Table of Contents
Introduction:
Heart disease continues to be a major cause of death worldwide in contemporary medicine. Heart valve dysfunction is a complex cardiovascular ailment that often necessitates surgical intervention to replace or repair the damaged valves. Historically, the procedure for heart valve replacement has typically utilized either mechanical valves or bioprosthetic valves sourced from animal tissues. Although these methods have been effective in saving several lives, they do have notable disadvantages, such as the requirement for lifetime anticoagulant therapy or limited long-term effectiveness. Consequently, there is an increasing fascination with the creation of highly sophisticated, biocompatible substances that cannot only substitute but also heal impaired heart valves. For this purpose, interactive double network (DN) hydrogels are engineered.
DN hydrogels are an advanced substance that can completely transform heart valve regeneration. The unique mechanical properties and biocompatibility of these hydrogels enable their precise imitation of the natural form and function of heart valves. This thorough investigation examines the scientific principles underlying DN hydrogels, their manipulation through engineering techniques, and their potential application in heart valve regeneration. A schematic overview of the fabrication process, structure, and function of the DHVs coated with PEG/PSBMA double network hydrogel (PEG/PSBMA-DHV).
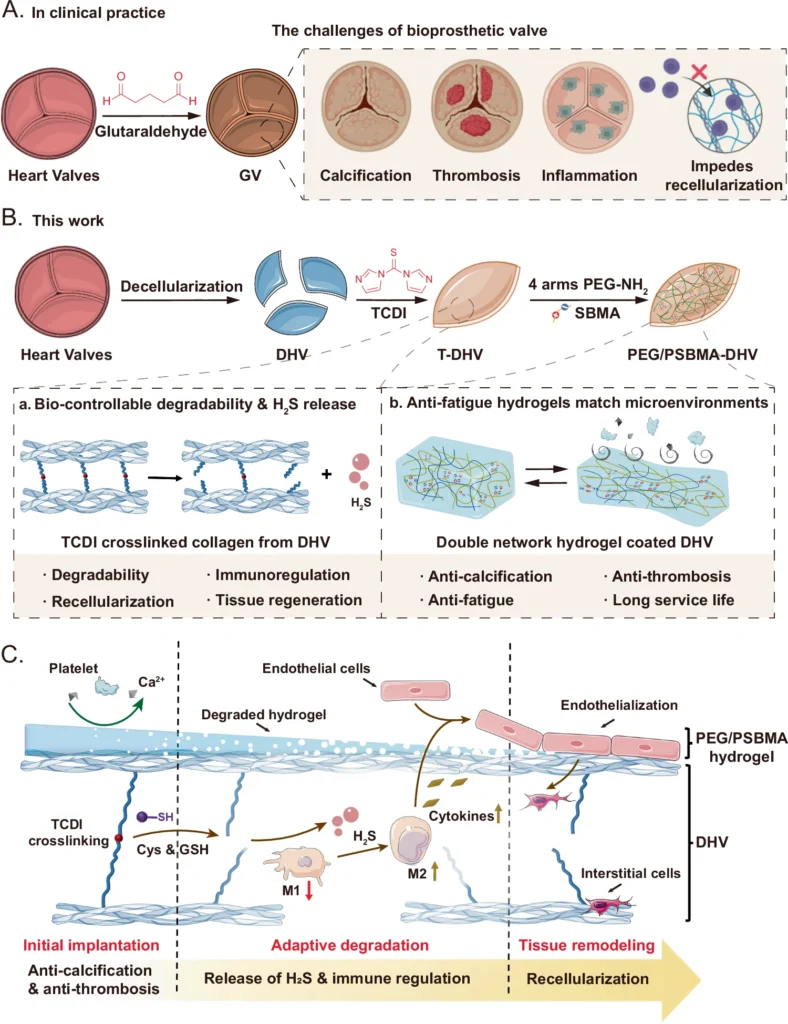
Hydrogels in Biomedical Applications: An Exploration
Before delving into the details of DN hydrogels, it is crucial to grasp the overarching classification of hydrogels and their importance in biomedical contexts. Hydrogels are polymer networks with a three-dimensional structure that can absorb and retain significant quantities of water. Hydrogels, while having a large amount of water, can preserve their structure, which makes them highly suitable for a wide range of biomedical uses.
Hydrogels are highly important in tissue engineering due to their capacity to imitate the extracellular matrix (ECM), which is an intricate network of proteins and carbohydrates that offer structural support to cells in the body. Hydrogels possess an ECM-like characteristic that enables them to facilitate cell adhesion, proliferation, and differentiation, which are crucial for tissue regeneration.
Hydrogels possess a high degree of tunability, allowing for precise adjustments to their physical and chemical characteristics to meet specific application requirements. By modifying the polymer network’s composition, one can control the stiffness, porosity, and breakdown rate of hydrogels. Due to their versatile nature, hydrogels find widespread use in various biomedical applications like drug delivery systems, wound dressings, and tissue scaffolds.
The Double Network Hydrogels Concept:
Double-network hydrogels are an advanced form of hydrogel technology that aims to address the drawbacks of conventional hydrogels. Two interpenetrating polymer networks, either chemically or physically connected, make up a Double Network Hydrogels. The initial network is generally a pliable and malleable polymer that offers both flexibility and biocompatibility. The second network typically consists of an inflexible and fragile polymer that provides mechanical durability and elasticity.
The amalgamation of these two networks yields a substance that demonstrates remarkable resilience and longevity, qualities that are especially crucial for applications involving mechanical strain, such as heart valves. The two networks work together to make DN hydrogels absorb energy more efficiently. This stops cracks from forming and increases the material’s overall strength.
DN hydrogels possess a distinctive set of characteristics that make them particularly well-suited for use in regenerative medicine. In this field, materials must not only facilitate cell development and tissue regeneration but also endure the mechanical stresses exerted by the human body. Construction of the TCDI crosslinked DHV (T-DHV) and the mechanism of H2S release.
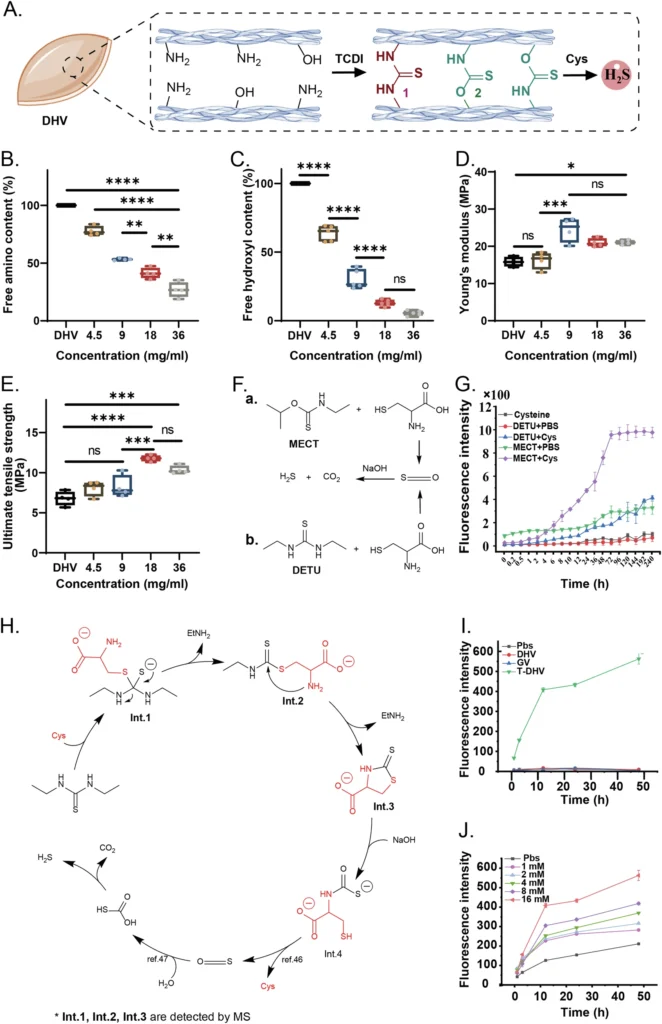
Effortless Engineering: An Innovative Approach
Facile engineering is the practice of creating intricate materials using simplified and effective methods. Within the realm of Double Network Hydrogels, facile engineering refers to the process of creating hydrogels with precise mechanical and biological characteristics by straightforward and repeatable techniques. This methodology differs from conventional methods of material synthesis, which are sometimes time-consuming, expensive, and challenging to expand.
The use of facile engineering provides numerous significant benefits in the development of Double Network Hydrogels. Initially, it facilitates the quick creation of hydrogels with diverse compositions and topologies, enabling researchers to promptly determine the most favorable candidates for particular applications. Furthermore, simple engineering techniques frequently require a smaller number of processes and lower temperatures, reducing the risk of harming delicate biological components. These technologies are both simple and scalable, which makes them more practical for large-scale production. This is particularly significant for clinical applications.
Characteristics of Interactive Double Network Hydrogels:
An intriguing characteristic of Double Network Hydrogels is their ability to interact with their environment. Double Network Hydrogels possess the ability to react to several stimuli, such as alterations in pH, temperature, or mechanical stress, unlike conventional hydrogels which are comparatively inert materials. Double Network Hydrogels’ ability to quickly and effectively respond to changes in their surroundings makes them ideal for use in dynamic situations such as the cardiovascular system.
As seen in heart valve regeneration, Double Network Hydrogels have interactive qualities that enable them to adjust to the continuous mechanical pressures applied by the beating heart. The capacity to adapt is essential for preserving the structural integrity of the engineered valve as time progresses. Additionally, Double Network Hydrogels can make themselves more compatible with living things by responding to environmental cues and either releasing bioactive substances or breaking down in response to certain physiological signals.
DN hydrogels are notable for their significant mechanical robustness. Heart valves experience substantial mechanical stresses during their cyclic opening and closing with each pulse. Therefore, any material used for valve replacement or regeneration must be able to withstand these forces without failure. Double Network Hydrogels possess an optimal combination of flexibility and durability, making them highly suitable for this particular task. Furthermore, we can intentionally design Double Network Hydrogels to undergo slow degradation, allowing the natural tissue to replace the scaffold during the regeneration process. Construction and characterization of PEG/PSBMA-DHV.
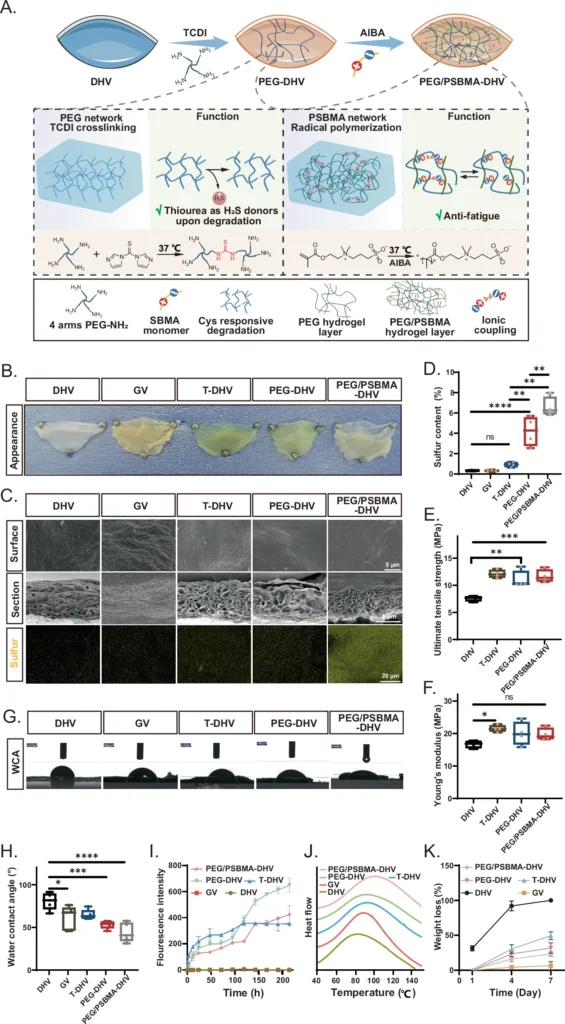
We are developing Double Network Hydrogels to aid in heart valve regeneration:
Heart valves are intricate formations that necessitate materials possessing highly specialized qualities. Many parameters, including the hydrogel’s mechanical characteristics, biocompatibility, and rate of degradation, must be considered in the effective design of a Double Network Hydrogels for heart valve regeneration.
Mechanical Properties: Double Network Hydrogels need to accurately replicate the mechanical characteristics of normal heart valves. This includes the material’s strength, durability, pliability, and resilience. For heart valves to function properly, they must be able to open and close smoothly throughout each pulse. Therefore, the hydrogel material used for heart valves must have the ability to withstand repeated deformation without compromising its structural integrity. To accomplish this, researchers can modify the composition and crosslinking density of the polymer networks. Additionally, they can introduce reinforcing agents such as nanoparticles or fibers.
Biocompatibility: Biocompatibility is a crucial consideration while designing Double Network Hydrogels for heart valve regeneration. The hydrogel must exhibit compatibility with the adjacent tissue and facilitate cellular development and proliferation. One way to accomplish this is by integrating bioactive chemicals, such as growth factors or peptides, into the hydrogel matrix. These chemicals can enhance cell adhesion and differentiation, which helps in the seamless integration of the designed valve with the surrounding tissue.
Decomposition Rate: The rate at which the Double Network Hydrogels decompose is also a crucial factor to take into account. Ideally, the Double Network Hydrogels should undergo degradation at a rate that aligns with the pace of tissue regeneration, providing transient support as the natural tissue grows. One way to manage this is by choosing polymers with varying rates of breakdown and altering the density of crosslinking in the hydrogel. In vitro hemocompatibility and biocompatibility of PEG/PSBMA-DHV.
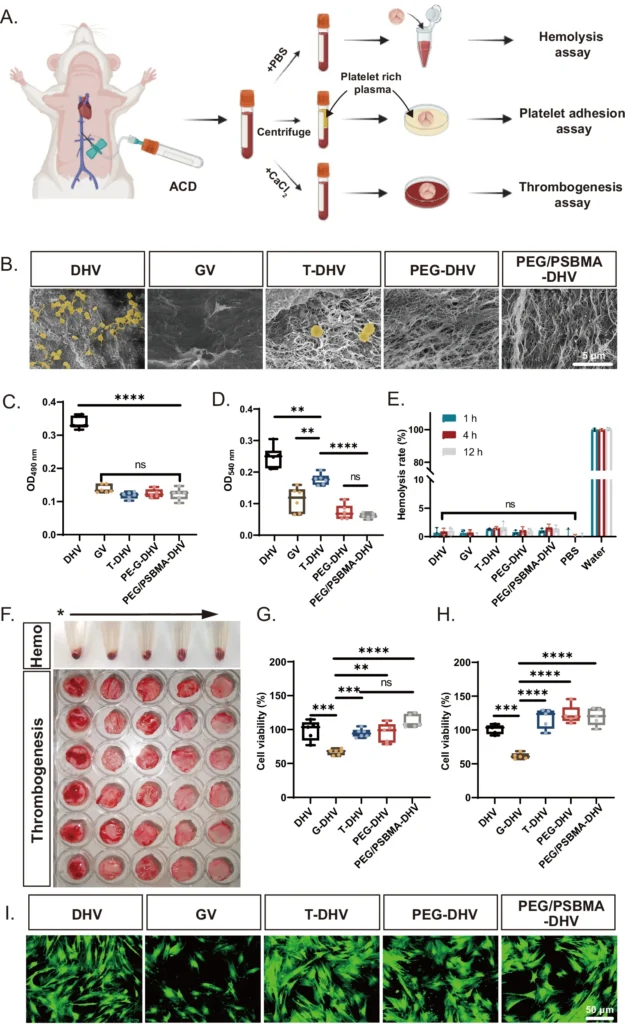
Materials used in Double Network Hydrogels for Heart Valve Regeneration:
The efficacy of Double Network Hydrogels in heart valve regeneration heavily depends on the selection of materials used in their fabrication. Double Network Hydrogels consist of two distinct polymer networks, each providing distinct properties to the resulting material.
First Network (Soft and Ductile): The first network within a Double Network Hydrogels typically consist of a pliable and malleable polymer that offers both flexibility and compatibility with living organisms. The network commonly utilizes polyacrylamide (PAAm), poly(ethylene glycol) (PEG), and natural polymers such as alginate or gelatin. People commonly select Polyacrylamide (PAAm) due to its elevated water content and adjustable mechanical characteristics. In contrast, natural sources yield alginate and gelatin, which have inherent bioactivity and are highly appealing for biomedical applications.
Second Network (Rigid and Brittle): A hard and brittle polymer contributes mechanical strength to the second network. This network typically consists of polymers such as poly(2-acrylamido-2-methylpropanesulfonic acid) (PAMPS) or poly(N-isopropyl acrylamide) (PNIPAM). PMPS, specifically, is renowned for its robust ionic interactions that enhance the durability of the hydrogel. PNIPAM, however, exhibits thermoresponsive behavior, allowing it to alter its characteristics in reaction to temperature fluctuations—an advantageous attribute for specific biomedical uses.
Reinforcing Agents: Double Network Hydrogels can benefit from the addition of reinforcing substances such as nanoparticles, nanofibers, or carbon-based materials like graphene to enhance their mechanical properties. These additions can enhance the strength, toughness, and elasticity of the hydrogel, hence increasing its suitability for usage in mechanically challenging situations such as heart valves. Evaluation of the immunomodulatory capacity of samples.
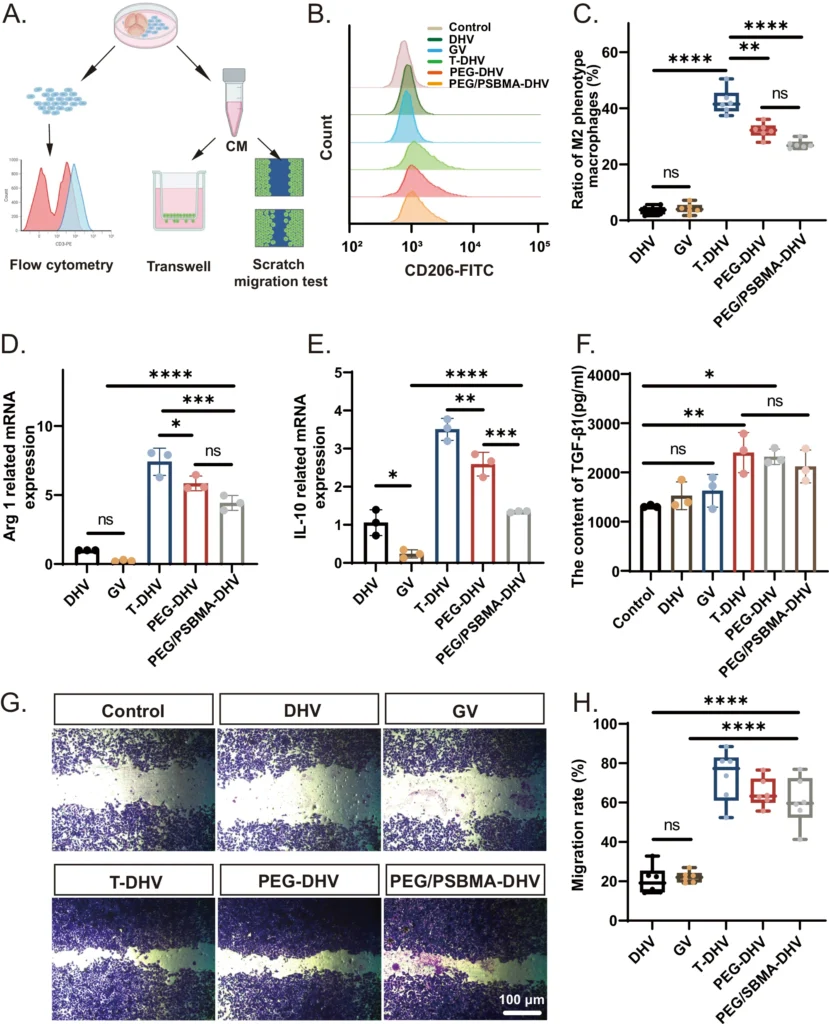
Methods for Creating Double Network Hydrogels:
The production of Double Network Hydrogels entails the meticulous assembly of two interpenetrating polymer networks. We employ various methods to create Double Network Hydrogels, each possessing distinct benefits and drawbacks.
Solution Casting: Solution casting is a commonly employed and straightforward technique for producing Double Network Hydrogels. This technique involves the initial formation of a polymer network by dissolving the polymer in a solvent and then pouring it into a mold to form a polymer network. We then create the second network by immersing the first network in a solution containing the monomer for the second polymer and then initiating polymerization. You can employ this relatively simple technique to create hydrogels with a diverse array of compositions and characteristics. Nevertheless, the hydrogels produced may not possess the exact manipulation of structure and morphology necessary for more intricate uses.
In Situ Polymerization: In situ, polymerization refers to the process of creating a hydrogel immediately within the body or in a designated area, such as a mold or scaffold. This technique is especially advantageous for fabricating hydrogels that require precise adherence to a certain form or arrangement, such as a cardiac valve. Photopolymerization, which uses light to trigger the polymerization process, and chemical crosslinking, which employs a chemical reaction to generate the hydrogel, are two methods for performing in situ polymerization. This technique enables enhanced manipulation of the ultimate form and composition of the hydrogel, although it may entail increased intricacy and time consumption compared to solution casting.
3D Bioprinting: 3D bioprinting is a developing method that enables exact manipulation of the structure and makeup of Double Network Hydrogels. Using a 3D printer, this technique applies successive layers of polymer solution, which then undergoes chemical bonding to form the hydrogel. This method enables the fabrication of intricate structures with meticulous regulation of the porosity, stiffness, and other characteristics of the hydrogel. Because of its ability to integrate cells and bioactive compounds into the hydrogel while printing, 3D bioprinting is highly advantageous for fabricating scaffolds in tissue engineering. Rapid endothelialization of the scaffolds under a hemodynamic environment.
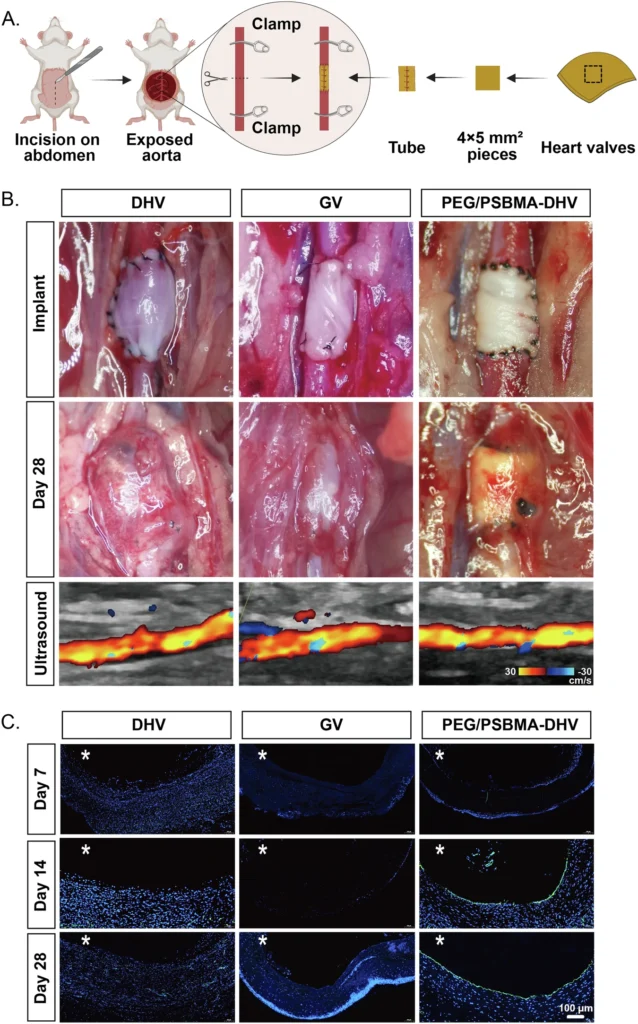
Double Network Hydrogels’ Mechanical Properties:
The mechanical characteristics of Double Network Hydrogels are crucial, especially when considering heart valve regeneration. The hydrogel used for valve replacement or regeneration must be able to withstand the continuous mechanical stresses exerted on heart valves during their cyclic opening and closing with each pulse without experiencing any structural failure.
Strength and Toughness: Double Network Hydrogels possess remarkable strength and toughness, which is one of their main advantages. Double Network Hydrogels have a unique composition, consisting of both a pliable and flexible network as well as a rigid and fragile network. This combination enables effective energy dissipation, reducing the spread of cracks and improving the overall strength of the material. Double Network Hydrogels are very suitable for applications that involve mechanical stress, such as heart valves.
Elasticity: Double Network Hydrogels not only possess strength and toughness but also demonstrate exceptional elasticity. This is crucial for heart valves, as they must possess the ability to undergo deformation and subsequently revert to their initial shape with every heartbeat. You can modify the flexibility of Double Network Hydrogels by manipulating the composition and crosslinking density of the polymer networks, and by adding reinforcing agents like nanoparticles or fibers.
Fatigue Resistance: Double Network Hydrogels provide a significant level of fatigue resistance, which is a key mechanical characteristic. Heart valves have several cycles of opening and closing over a person’s life. So, the hydrogel that is used to replace or grow a valve needs to be able to withstand repeated mechanical stress without breaking down. DN hydrogels have demonstrated exceptional resistance to fatigue, making them very suitable for extended utilization in the cardiovascular system.
Biological Evaluation of Double Network Hydrogels:
The biological performance of DN hydrogels is crucial for their application in heart valve regeneration, in addition to their mechanical qualities. DN hydrogels must exhibit biocompatibility, which refers to their ability to neither elicit an immune response nor induce injury to the adjacent tissue. Furthermore, they must promote cell adhesion and proliferation, promoting seamless integration of the designed valve with the surrounding host tissue.
Cell Adhesion and Proliferation: Cell adhesion and proliferation are crucial factors in tissue engineering, as they determine whether the designed scaffold effectively supports cell attachment and growth. Bioactive compounds, such as peptides or growth factors, can enhance DN hydrogels and facilitate cell adhesion and proliferation. During the production process, these molecules can either integrate into the hydrogel matrix or gradually release from it in response to specific physiological signals.
Biocompatibility: Biocompatibility is a crucial consideration while designing DN hydrogels for heart valve regeneration. The hydrogel must exhibit compatibility with the adjacent tissue and must not elicit an immune response or induce injury to the host. You can achieve this by selecting polymers that are naturally compatible with living organisms, like alginate or gelatin, or by modifying the hydrogel’s surface to reduce its potential to trigger an immune response.
Decomposition Rate: The rate at which the DN hydrogel decomposes is also a crucial factor to consider. Optimally, the hydrogel should undergo degradation at a rate that aligns with the pace of tissue regeneration, offering temporary reinforcement while the native tissue undergoes growth. One way to regulate this is by selecting polymers with varying degradation profiles and adjusting the density of crosslinking in the hydrogel.
Engineering DN hydrogels presents several challenges:
Although DN hydrogels have numerous benefits, their engineering is not devoid of difficulties. A significant challenge lies in achieving consistency in the hydrogel’s characteristics, particularly when expanding manufacturing for clinical applications. DN hydrogels consist of two interpenetrating polymer networks, making it challenging to achieve uniform distribution of these networks within the hydrogel. These differences in the mechanical and biological properties of the hydrogel can impact its performance in living organisms.
Achieving Uniformity: To tackle this difficulty, researchers are working on innovative fabrication processes that enable enhanced regulation of the polymer networks’ distribution, thus achieving uniformity. 3D bioprinting enables the accurate placement of polymer solutions, facilitating the production of hydrogels with consistent characteristics. In addition, scientists are investigating the application of reinforcing elements, such as nanoparticles or fibers, to enhance the consistency of the hydrogel.
Balancing Mechanical Strength and Biocompatibility: One of the challenges in developing DN hydrogels is finding the right balance between mechanical strength and biocompatibility. The second network in a DN hydrogel often consists of a stiff and brittle polymer, which imparts mechanical strength but may be less biocompatible and more challenging to degrade compared to the first network. To tackle this problem, scientists are investigating the use of novel polymers and crosslinking agents that can enhance the biocompatibility of the secondary network without compromising its mechanical robustness.
Expanding manufacturing: Expanding the manufacturing of DN hydrogels for clinical application poses an additional noteworthy obstacle. Conventional techniques for creating hydrogels, such as solution casting or in situ polymerization, can be laborious and challenging to expand for mass production. To tackle this difficulty, scientists are investigating novel fabrication techniques, like 3D bioprinting or microfluidic approaches, that enable the quick and efficient synthesis of DN hydrogels on a large scale.
Applications in Clinical Settings and Stories of Achievement:
Various studies are currently investigating the potential of DN hydrogels in heart valve regeneration. Preliminary clinical trials have shown encouraging results in using DN hydrogels to replace or restore impaired heart valves. These hydrogels have displayed exceptional mechanical characteristics, biocompatibility, and assimilation with the surrounding tissue.
Case Studies: A notable example of success is the use of DN hydrogels in the process of regenerating aortic valves. Researchers recently successfully implanted a DN hydrogel-based aortic valve in a patient with aortic stenosis, a disorder characterized by the narrowing of the aortic valve and subsequent restriction of blood flow. The hydrogel-based valve exhibited exceptional mechanical capabilities, enduring the continuous mechanical forces exerted by the cardiovascular system without experiencing any failures. In addition, the valve exhibited excellent integration with the host tissue, showing no indications of immunological rejection or any negative consequences.
Current Progress and Future Prospects: The success of this and subsequent experiments has sparked considerable interest in utilizing DN hydrogels for heart valve regeneration, both in terms of current progress and prospects. Scientists are also investigating the application of DN hydrogels in additional heart valve types, such as the mitral or tricuspid valves, as well as in other areas of regenerative medicine. DN hydrogels are now under investigation for their potential use in cartilage regeneration, wound healing, and drug administration systems. These hydrogels provide a distinctive blend of mechanical strength, flexibility, and biocompatibility, making them highly valuable in these areas. Overall performance of the samples after 4-week implantation.
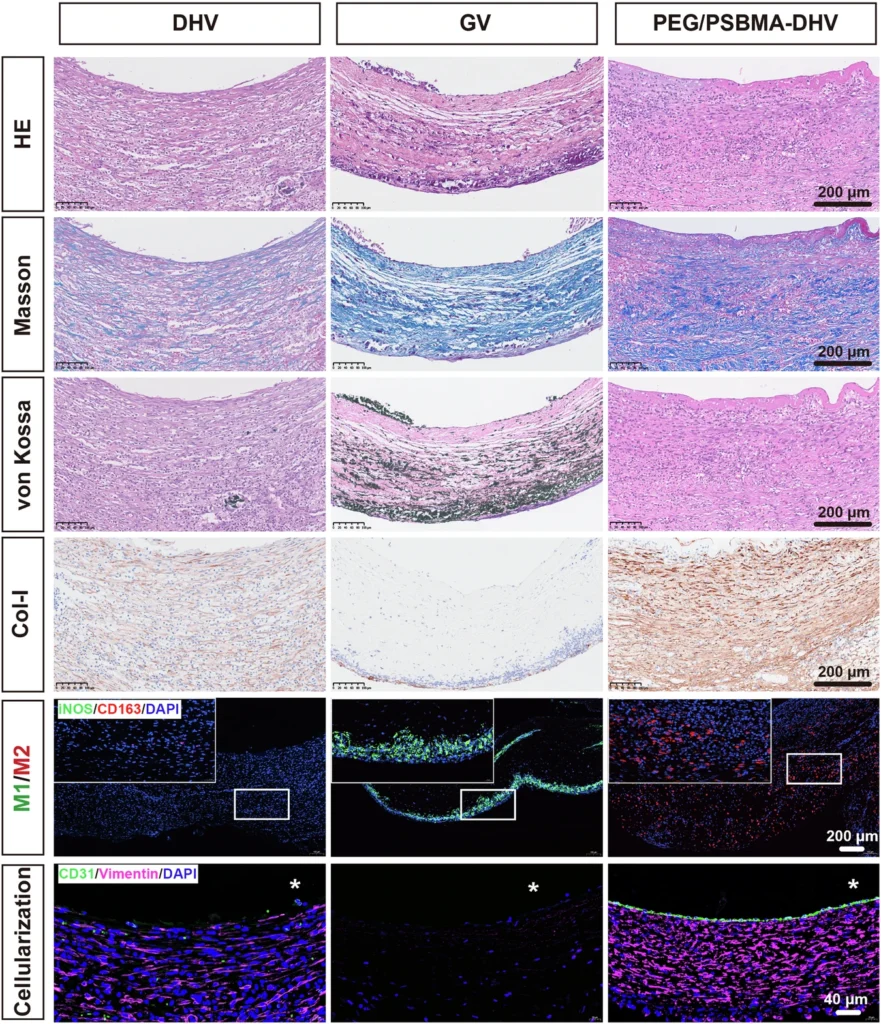
We are conducting a comparative analysis of biomaterials with other materials:
When compared to other biomaterials used in heart valve creation, DN hydrogels possess a variety of unique advantages. An important benefit is their capacity to integrate both mechanical robustness and pliability within a single substance. Conventional materials used in the construction of heart valves, such as mechanical valves or bioprosthetic valves, frequently necessitate trade-offs between durability and pliability. However, it is possible to design DN hydrogels to have both durability and pliability, making them a more adaptable and efficient substance for cardiac valve regeneration.
Cost-Effectiveness and Scalability: DN hydrogels have the advantage of being both cost-effective and scalable. Conventional approaches to heart valve replacement, such as mechanical valves or bioprosthetic valves, can be costly and challenging to expand for wider adoption. On the other hand, simple and cost-effective techniques can create DN hydrogels, making them a more viable option for large-scale production. Furthermore, the capacity of DN hydrogels to scale up makes them ideal for use in environments with limited resources, where access to advanced medical technology may be restricted.
Long-Term Durability: The lack of long-term durability limits traditional cardiac valve replacement methods. Mechanical valves require long-term anticoagulation therapy to prevent the formation of blood clots, increasing the risk of bleeding and other complications. Bioprosthetic valves, although they are more compatible with living tissue, have a finite lifespan and may require replacement after a few years. DN hydrogels have the potential to be durable over a long period without requiring anticoagulant medication. This makes them a more appealing choice for regenerating hearts.
Future Prospects for Heart Valve Regeneration Using Hydrogel-Based Techniques:
The prospects for DN hydrogels in heart valve regeneration are promising. Scientists are investigating novel combinations of polymers, strategies for creating hydrogels, and methods for adding functionality to improve the qualities of these materials. The primary objective is to develop a completely operational, bioengineered cardiac valve that may be surgically implanted with low risk and provide maximal long-term advantages to patients.
Potential Innovations in Hydrogel Engineering: Researchers are currently exploring smart hydrogels to advance hydrogel engineering. These hydrogels can react to environmental changes, such as variations in pH, temperature, or mechanical stress. The development of heart valves that can adapt to the dynamic conditions of the circulatory system could potentially leverage these intelligent hydrogels, thereby enhancing their long-term effectiveness and resilience.
Long-Term Goals and Research Goal Areas: Another research goal is the advancement of hydrogels with the ability to facilitate the regeneration of cardiac tissue, rather than solely substituting it. Researchers aim to enhance the growth and repair of injured tissue by integrating bioactive molecules, such as growth factors or stem cells, into the hydrogel matrix. We expect this approach to lead to the development of cardiac valves that can actively facilitate the regeneration process, resulting in more comprehensive and long-lasting tissue restoration.
Potential Consequence: In the future, DN hydrogels could have a significant impact on heart valve regeneration. With the ongoing advancement of technology, DN hydrogels have the potential to significantly alleviate the impact of cardiovascular disease and enhance the well-being of numerous individuals. DN hydrogels offer a resilient, compatible with living organisms, and economical option for replacing heart valves, which can contribute to meeting the increasing need for sophisticated medical technologies in an aging society.
In conclusion:
To summarize, the easy manipulation of interactive DN hydrogels is a notable advancement in the field of heart valve regeneration. These materials provide a potent amalgamation of mechanical robustness, adaptability, and compatibility with living organisms, rendering them highly suitable for the advancement of heart valves in the next era. DN hydrogels have the potential to significantly improve the treatment of heart valve disease and enhance the quality of life for patients globally by offering a more resilient and biocompatible alternative to conventional heart valve replacement procedures.
The transition from laboratory experimentation to practical implementation in clinical settings is a lengthy process, but the advancements achieved thus far are encouraging. As researchers further investigate novel materials, production methods, and applications, DN hydrogels will certainly have a growing significance in the field of regenerative medicine. We expect DN hydrogels to take the lead in the promising field of heart valve regeneration.
Frequently Asked Questions:
1. What are the primary benefits of DN hydrogels for heart valve regeneration?
DN hydrogels possess a distinctive blend of robustness, pliability, and compatibility with living organisms, rendering them well-suited for the regeneration of heart valves. Their capacity to imitate the inherent characteristics of heart valves while offering both long-lastingness and flexibility is a notable advantage.
2: How does DN hydrogels’ mechanical robustness compare to that of native heart valves?
We can design DN hydrogels to match or exceed the mechanical strength of natural heart valves. DN hydrogels have interpenetrating polymer networks that allow them to efficiently lose energy. This stops cracks from spreading and makes the material stronger overall.
3. What are the primary obstacles encountered in the development of DN hydrogels?
The primary obstacles to the development of DN hydrogels include achieving homogeneity in the hydrogel’s characteristics, striking a balance between mechanical robustness and biocompatibility, and expanding production capacity for clinical applications. The advancement of novel production techniques, exploration of polymer combinations, and utilization of reinforcing agents are addressing these issues.
4. Are DN hydrogels considered biocompatible for extended periods within the human body?
Indeed, we specifically engineer DN hydrogels to possess biocompatibility and biodegradability, ensuring their safety for extended periods of use. We can regulate the breakdown rate of DN hydrogels to match the rate of tissue regeneration, ensuring the material provides temporary support while the natural tissue develops
5. What are the potential applications of DN hydrogels in other regenerative medicine domains?
In addition to heart valve regeneration, DN hydrogels possess prospective uses in diverse fields of regenerative medicine, such as cartilage repair, wound healing, and drug delivery systems. Due to their distinctive blend of mechanical robustness, adaptability, and ability to interact harmoniously with living organisms, they are an adaptable substance suitable for a diverse array of biomedical uses.
For more chemistry blogs, visit chemistry Master