Table of Contents
Preface of Cycloaddition of Bicyclobutanes:
Enantioselectivity is fundamental in contemporary organic chemistry, particularly in pharmaceuticals, where molecular chirality can influence medication efficacy or toxicity. In organic synthesis, attaining high enantioselectivity frequently presents a problem, especially when addressing complicated compounds with many chiral centers. The enantioselective formal (3 + 3) cycloaddition of bicyclobutanes with nitrones has garnered much attention. With the help of asymmetric Lewis acids, this reaction is a promising way to make highly selective molecules for one enantiomer. These molecules can be used in drug research and other complex chemical tasks.
The use of bicyclobutanes as reactants is particularly intriguing because of their strained ring architecture, which makes them highly reactive and suitable for cycloaddition processes. In the process, nitrones function as 1,3-dipoles, facilitating the formation of intricate heterocyclic structures. When catalyzed by an asymmetric Lewis acid, this reaction yields enantiomerically pure compounds, rendering it particularly advantageous for synthetic chemists.
This essay will examine the complexities of this reaction, emphasizing its mechanism, problems, and applications. In the end, you’ll have a deep understanding of how the enantioselective formal (3+3) cycloaddition of bicyclobutanes with nitrones can be used to make useful chemicals very precisely and quickly. Outline of this work.
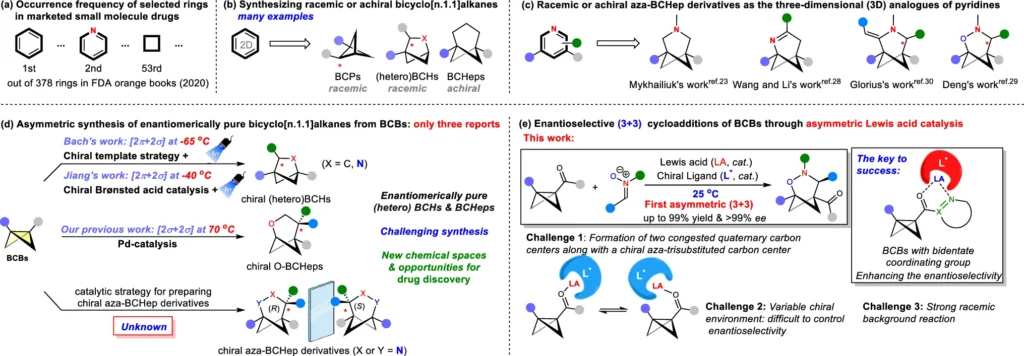
Comprehending cycloaddition reactions:
Cycloaddition reactions are essential in organic chemistry. These reactions entail the amalgamation of two or more unsaturated molecules to create a cyclic structure. This transition is critical in the synthesis of complex compounds, particularly those with ring structures, which are common in natural products and pharmaceuticals. The number of atoms participating in the formation of the new ring often categorizes cycloaddition reactions. A (2+2) cycloaddition entails the fusion of two molecules, in which two atoms from each reactant contribute to the formation of a four-membered ring.
The formal (3+3) cycloaddition, which is the subject of this article, is a variant of the conventional cycloaddition in which two reactants each donate three atoms to form a six-membered ring. These reactions, despite being less prevalent than (2+2) or (4+2) cycloadditions, are highly advantageous for the synthesis of intricate six-membered ring structures. These systems are crucial in pharmaceutical production, as six-membered rings frequently contribute significantly to the compound’s biological activity.
The Formal (3 + 3) Cycloaddition:
A formal (3 + 3) cycloaddition denotes a reaction in which three atoms from one reactant and three atoms from another reactant unite to create a new six-membered ring. This does not constitute a conventional coordinated cycloaddition akin to the Diels-Alder process, which entails a (4 + 2) cycloaddition wherein six atoms generate a new ring in a singular step. The formal (3 + 3) process often entails several stages, during which intermediates are generated before the ultimate ring closure.
The formal (3+3) cycloaddition of bicyclobutanes and nitrones is particularly advantageous because it facilitates the synthesis of intricate ring structures with multiple chiral centers. The level of complexity is hard to achieve using other synthetic methods, which makes the (3+3) cycloaddition a very effective way to make these compounds. An asymmetric Lewis acid can also help the reaction go more smoothly, which lets one enantiomer be made more easily than the other. This is very important for drug development. Scope I: variation of the nitrones.
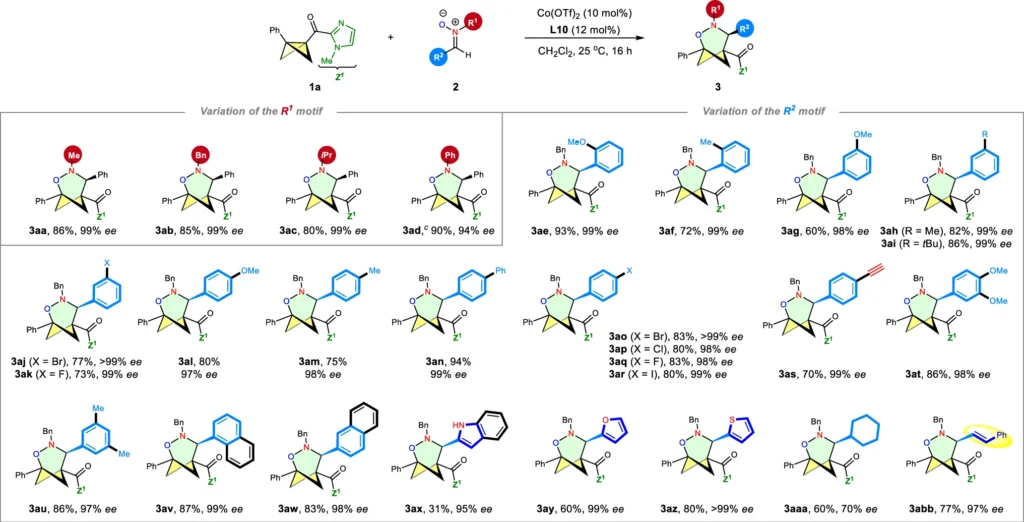
Bicyclobutanes: An Exceptional Structural Component
Bicyclobutanes are intriguing compounds because of their distinctive structure. They comprise two fused four-membered rings, resulting in considerable ring tension. This strain arises because the bond angles in a four-membered ring are significantly smaller than the ideal 109.5° angles characteristic of sp3 hybridized carbon atoms. Bicyclobutanes exhibit high reactivity due to their propensity to alleviate tension via bond-breaking and rearrangement events.
The elevated reactivity of bicyclobutanes makes them ideal reactants for cycloaddition processes, where ring strain alleviation facilitates the formation of new bonds. When nitrones are added to bicyclobutane in the formal (3+3) cycloaddition, the strain is relieved as the ring opens up to make a new six-membered ring. This facilitates the effective construction of intricate molecular architectures, which are crucial in numerous domains of organic synthesis.
The application of bicyclobutanes in cycloaddition reactions has increased in popularity as scientists recognize their versatility and reactivity. Chemists can exploit bicyclobutanes’ reactivity to synthesize intricate and anti-selective compounds by meticulously regulating reaction conditions and applying appropriate catalysts.
Nitrones in Organic Chemistry:
Nitrones represent a significant category of chemicals in organic synthesis. They are defined by a nitrogen-oxygen (N=O) double bond next to an alkyl or aryl group. Nitrones act as 1,3-dipoles in cycloaddition reactions, which lets them react with alkenes that don’t have enough electrons or strained substances like bicyclobutanes to make new ring structures.
In the formal (3+3) cycloaddition, nitrones react with bicyclobutanes to make heterocyclic rings with six members. This reaction is particularly advantageous in synthetic chemistry because it enables the creation of intricate ring structures that would be difficult to synthesize using alternative approaches. Nitrones are exceptionally versatile and can yield a diverse array of products, depending on the exact reaction conditions and reactants used.
Nitrones are important in enantioselective synthesis because they can participate in reactions that generate numerous chiral centers. Their value lies in the synthesis of physiologically active molecules, where careful control of stereochemistry is typically crucial to the molecule’s success. Scope II: variation of the BCBs
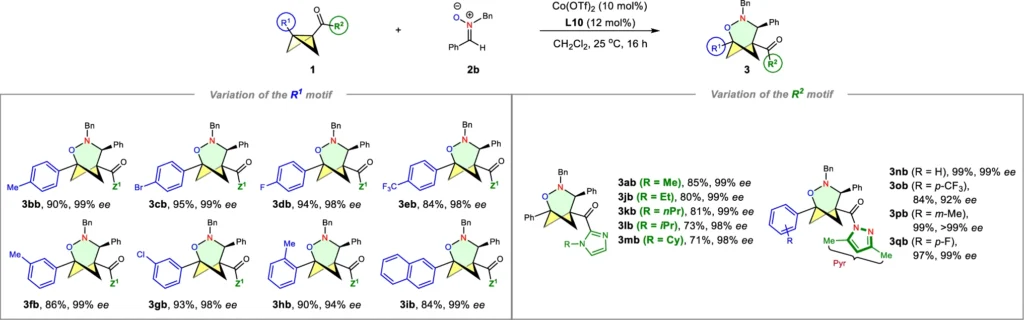
Enantioselectivity and Chirality:
Chirality is a crucial principle in chemistry, especially for organic synthesis. You cannot superimpose a chiral molecule on its mirror counterpart, just as you cannot completely align your left and right hands, which are mirror images of each other. This feature is significant in chemistry because numerous biological compounds are chiral, and a molecule’s chirality can substantially influence its biological activity.
Enantioselectivity denotes the preferential synthesis of one enantiomer relative to another in a chemical process. This is especially significant in pharmaceutical science, as the two enantiomers of a chiral medication may exert markedly distinct effects on the body. One enantiomer may confer therapeutic advantages, while the other may be inert or potentially detrimental.
In the formal (3+3) cycloaddition of bicyclobutanes and nitrones, strong enantioselectivity is required. Because chemists can choose to synthesize one enantiomer over its counterpart, they can make molecules that work as intended in living things while lowering the risk of side effects.
Lewis Acid Catalysis in Organic Reactions:
Lewis acids are essential in catalyzing certain organic processes, such as cycloadditions. A Lewis acid is defined as a chemical that can accept an electron pair, making it an electron-deficient entity. Organic chemistry frequently employs Lewis acids to activate electrophilic substrates, thereby enhancing their reactivity and promoting bond formation.
Lewis acids can coordinate with one or more reactants in cycloaddition processes, stabilizing transition states or intermediates and reducing the reaction’s activation energy. This facilitates a more seamless and efficient reaction. Prevalent Lewis acids used in organic synthesis include metal halides such as aluminum chloride (AlCl3) and boron trifluoride (BF3), as well as metal complexes like titanium tetrachloride (TiCl4).
Asymmetric Catalysis: Generating Antioxide Selectivity
When it comes to organic synthesis, asymmetric catalysis works well because it makes it easier to make one enantiomer over the other. To establish a chiral environment for the reacting molecules, asymmetric catalysis employs a chiral catalyst. The chemical environment influences the process, resulting in the preferential formation of one enantiomer.
Asymmetric Lewis acid catalysis is used to get high enantioselectivity in the formal (3+3) cycloaddition of bicyclobutanes and nitrones. The Lewis acid reacts with the nitrone and bicyclobutane, shifting them in a way that makes a certain transition state easier to reach. This state makes one enantiomer more likely to form than the other.
This form of asymmetric catalysis is very significant in pharmaceutical chemistry, as the chirality of a molecule can influence its medicinal efficacy. Chemists can produce enantiomerically pure compounds with the desired biological activity by employing an asymmetric catalyst. Scale-up and derivatizations.
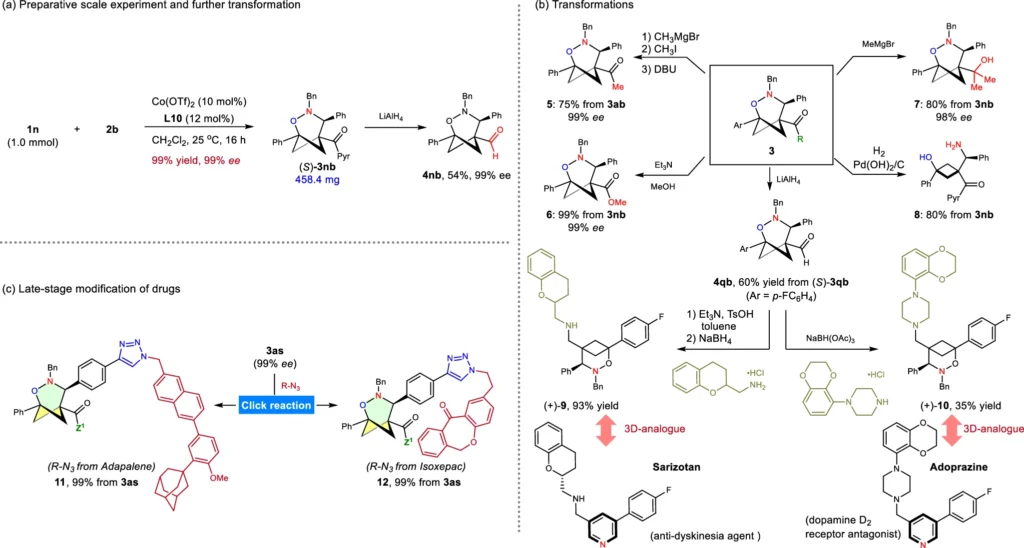
Mechanism of Bicyclobutanes and Nitrones Cycloaddition (3+3):
The formal (3+3) cycloaddition process, which involves bicyclobutanes and nitrones, occurs in multiple stages. The Nitron first coordinates with the Lewis acid catalyst, thereby enhancing its electrophilicity. This enables the bicyclobutane to engage with the nitrone and commence the cycloaddition reaction.
During the reaction, the strained bicyclobutane experiences a ring-opening process that alleviates the strain and facilitates the formation of new bonds between the nitrone and the bicyclobutane. The outcome is a six-membered ring featuring several novel chiral centers. The asymmetric Lewis acid catalyst creates a chiral environment that makes sure the reaction goes through a unique transition state, which leads to the production of one enantiomer more than the other.
Obstacles to Attaining Elevated Enantioselectivity:
It is hard to get high enantioselectivity in the formal (3+3) cycloaddition of bicyclobutanes and nitrones because there are so many factors that can go wrong. A primary challenge is regulating the stereochemistry of the process’s stereochemistry is a major challenge. Minor changes in reaction parameters, such as temperature or solvent, can have a significant impact on the process’s enantiomeric result.
The Lewis acid catalyst selection is essential. Some Lewis acids are better than others at helping with enantioselective reactions, and the enantioselectivity of the reaction is greatly affected by the presence or absence of chiral ligands on the catalyst. The reactants’ steric and electronic characteristics can influence the reaction’s enantioselectivity.
Chemists frequently must meticulously tune reaction conditions, experimenting with various catalysts, solvents, and temperatures to attain the appropriate enantioselectivity.
Lewis acid catalysts are selected for the reaction:
The Lewis acid catalyst selection in the formal (3+3) cycloaddition of bicyclobutanes and nitrones is critical for achieving elevated enantioselectivity. A variety of Lewis acids, such as metal halides and metal complexes, have been employed in this reaction type. The exact Lewis acid used could have a significant impact on the reaction’s results, particularly in terms of enantioselectivity and yield.
Enantioselective cycloaddition processes frequently employ metal complexes like titanium tetrachloride (TiCl4) and zinc triflate (Zn(OTf)2) as Lewis acid catalysts. To establish a chiral environment surrounding the reacting molecules and facilitate the preferential production of one enantiomer over the other, one can pair these catalysts with chiral ligands.
The Lewis acid catalyst selection also influences the reaction’s efficacy. Certain catalysts may accelerate processes or enhance yields, while others may lead to diminished enantioselectivity or generate undesirable byproducts. Consequently, it is crucial to meticulously choose the suitable catalyst according to the particular demands of the reaction. Non-linear effect study and DFT calculations.
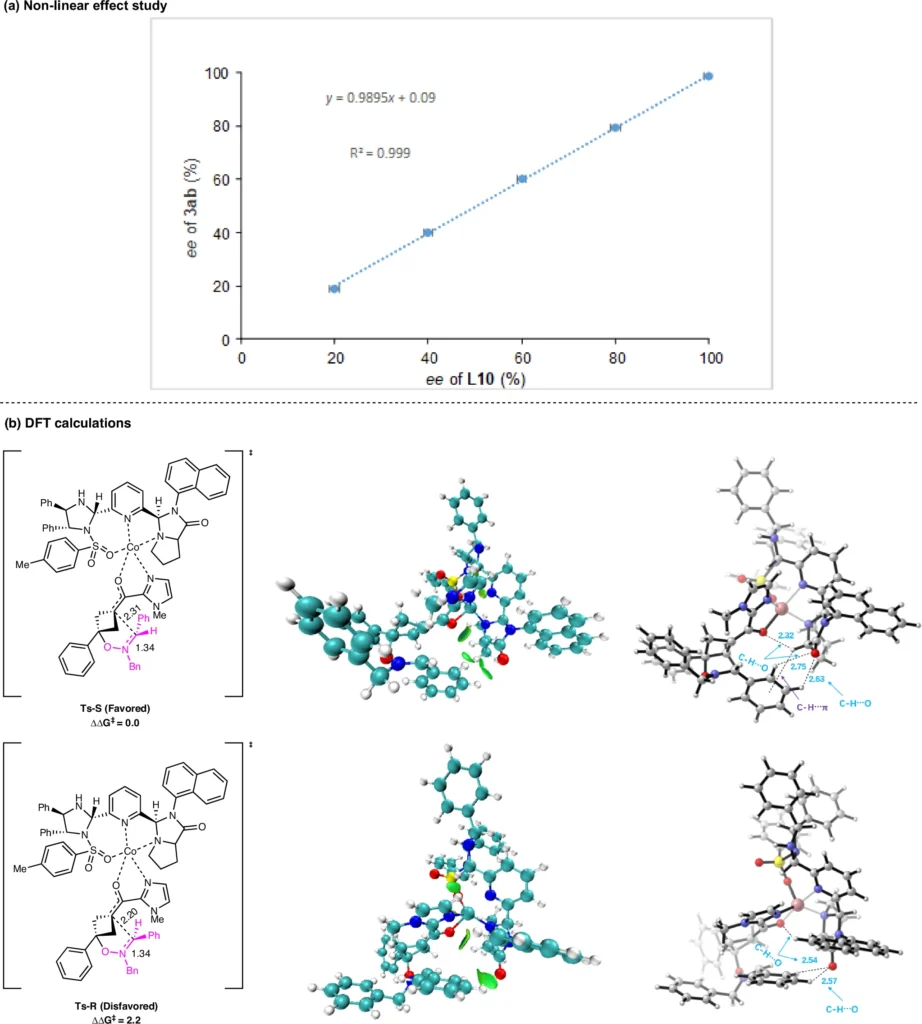
Conditions for Enantioselective (3 + 3) Cycloaddition Experiments:
The experimental parameters for the enantioselective formal (3+3) cycloaddition involving bicyclobutanes and nitrones require meticulous regulation to achieve high yields and enantiomeric excess. Multiple parameters, such as solvent selection, temperature, and reactant ratio, can profoundly influence the reaction’s outcome.
In cycloaddition processes, solvents like dichloromethane (CH2Cl2) or toluene are often used because they can dissolve the reactants and make the reaction environment stable. The solvent selection can affect the solubility of the Lewis acid catalyst and the stability of the reaction intermediates.
Temperature constitutes another essential variable. Lower temperatures often improve enantioselectivity by slowing down the reaction. This lets the chiral catalyst have a bigger impact on the reaction pathway. Nevertheless, lower temperatures can result in lower reaction rates, potentially reducing the overall reaction yield.
The ratio of reactants must be meticulously optimized. In numerous instances, an abundance of one reactant (such as nitrone) is employed to propel the reaction to completion and enhance yields.
Reaction Yields and Enantiomeric Excess:
The formal (3+3) cycloaddition’s efficacy is determined by both the reaction yield and the enantiomeric excess (ee) of the resultant product. Yield denotes the quantity of product acquired about the theoretical maximum, whereas enantiomeric excess quantifies the extent of selectivity for one enantiomer compared to the other.
In a highly enantioselective reaction, the enantiomeric excess generally exceeds 90%, indicating that more than 90% of the result consists of a single enantiomer. Getting better at enantioselectivity is important for making medicines because even small amounts of the wrong enantiomer can cause bad side effects.
Multiple factors affect both the yield and the enantiomeric excess of the reaction. This includes selecting the Lewis acid catalyst, the reaction parameters, and the reactants’ steric and electronic characteristics. Chemists can get higher yields and enantiomeric excess by carefully optimizing these variables. This makes the reaction more efficient and scalable for industrial use.
Comparison with Alternative Enantioselective Cycloaddition Reactions:
One type of enantioselective cycloaddition used in chemical synthesis is the formal (3+3) cycloaddition of bicyclobutanes and nitrones. Additional prevalent enantioselective cycloadditions encompass the (4 + 2) Diels-Alder reaction and (2 + 2) cycloadditions, both of which are extensively employed in the synthesis of intricate ring structures.
In comparison to alternative processes, the (3 + 3) cycloaddition presents numerous advantages. Initially, it facilitates the synthesis of six-membered rings containing numerous chiral centers in a singular process. This represents a considerable benefit in the synthesis of complicated molecules, where numerous steps are frequently necessary to establish chiral centers.
Nonetheless, the (3 + 3) cycloaddition presents certain obstacles. Getting higher enantioselectivity in this reaction can be harder than in other cycloadditions. This is mostly because the bicyclobutane substrate is very reactive. The selection of a Lewis acid catalyst is crucial, as not all catalysts exhibit equivalent efficacy in this process.
Applications in Pharmaceutical Chemistry:
There is a lot of potential in medicinal chemistry for the enantioselective products of the formal (3+3) cycloaddition involving bicyclobutanes and nitrones. This reaction’s capacity to synthesize complex, enantiomerically pure compounds in one step renders it exceptionally helpful for pharmaceutical research.
Numerous pharmaceuticals possess chiral centers, and the capacity to regulate the stereochemistry of these compounds is essential for their biological efficacy. The distinction between the two enantiomers of a medication can determine the disparity between therapeutic efficacy and toxicity. Scientists can make the target enantiomer very accurately by using enantioselective cycloaddition processes. This reduces side effects and improves the effectiveness of medicines.
In addition to pharmaceuticals, this reaction’s byproducts may have applications in agrochemicals and materials research. For the development of advanced materials and bioactive substances, the ability to make complex, functionalized molecules with high enantioselectivity opens up new possibilities.
Prospective Trajectories and Possible Enhancements:
An ongoing study on enantioselective cycloadditions reveals multiple avenues for enhancement. A possible avenue for enhancement is the development of novel Lewis acid catalysts that exhibit greater efficacy in facilitating enantioselectivity. By creating novel catalysts featuring enhanced chiral ligands, scientists can augment the efficiency and selectivity of reactions, rendering them more viable for industrial applications.
A future study should focus on broadening the range of reactants applicable in the formal (3 + 3) cycloaddition. Bicyclobutanes and nitrones have been shown to work well as reactants. Other strained molecules or dipoles may also be able to participate in this reaction, which increases the possibilities for molecular synthesis.
Ultimately, there exists the possibility to scale the reaction for industrial applications. Although the formal (3+3) cycloaddition demonstrates significant potential in laboratory settings, scaling the reaction for the production of substantial quantities of product remains a challenge. Optimizing the reaction conditions and catalyst systems may facilitate overcoming these hurdles, rendering the process more viable for large-scale production.
Final Assessment:
A big step forward in modern chemical synthesis is the enantioselective formal (3+3) cycloaddition of bicyclobutanes with nitrones, which is made easier by asymmetric Lewis acid catalysis. This reaction facilitates the efficient synthesis of complex, enantiomerically pure compounds in a single step, rendering it highly advantageous for applications in pharmaceuticals, materials science, and other fields.
Notwithstanding the difficulties in attaining high enantioselectivity and refining reaction conditions, the promise of this reaction is evident. As research progresses and novel catalysts and methodologies emerge, the formal (3 + 3) cycloaddition is expected to become an increasingly vital instrument in the chemist’s repertoire.
Frequently Asked Questions:
1). Why is enantioselectivity important in the synthesis process?
Enantioselectivity ensures the preferential formation of one enantiomer over the other, which is critical in medicine due to the significantly varied biological effects exhibited by distinct enantiomers.
2). In what manner do Lewis acids facilitate cycloaddition reactions?
Lewis acids take electron pairs, which stabilizes transition states and lowers activation energies. This speeds up cycloaddition processes and makes them more efficient.
3). Under these conditions, what accounts for the reactivity of bicyclobutanes?
The ring structure of bicyclobutanes is very strained, so they get rid of this strain through reactions that make bond breaking easier and the formation of more stable structures.
4). What are the standard yields in enantioselective cycloadditions?
In enantioselective cycloadditions, yields can change. But in the formal (3+3) cycloaddition of bicyclobutanes with nitrones, yields are usually high, with enantiomeric excesses exceeding 90%.
5). What impact does this strategy have on drug development?
This technique facilitates the efficient synthesis of enantiomerically pure compounds, which is essential in pharmaceutical development. Enantiopure pharmaceuticals frequently exhibit superior efficacy and fewer adverse effects compared to racemic mixes.
For more chemistry blogs, visit chemistry Master