Table of Contents
Overview of Electron Transfer Dynamics:
Electron transfer is a fundamental mechanism in chemistry and physics, crucial for biological systems, organic electronics, and energy conversion. Electron transfer occurs over many periods, ranging from milliseconds to several femtoseconds (one femtosecond equals 10⁻¹⁵ seconds). Recently, researchers have concentrated on ultrafast electron transfer kinetics in specifically engineered molecular systems, such as donor-acceptor molecules. These compounds are intriguing because of their capacity to absorb light and facilitate electron transfer across chemical structures in remarkably brief intervals, frequently within a few microseconds. What is its significance, and what are the implications for science and technology? Molecular structures and experimental strategy.
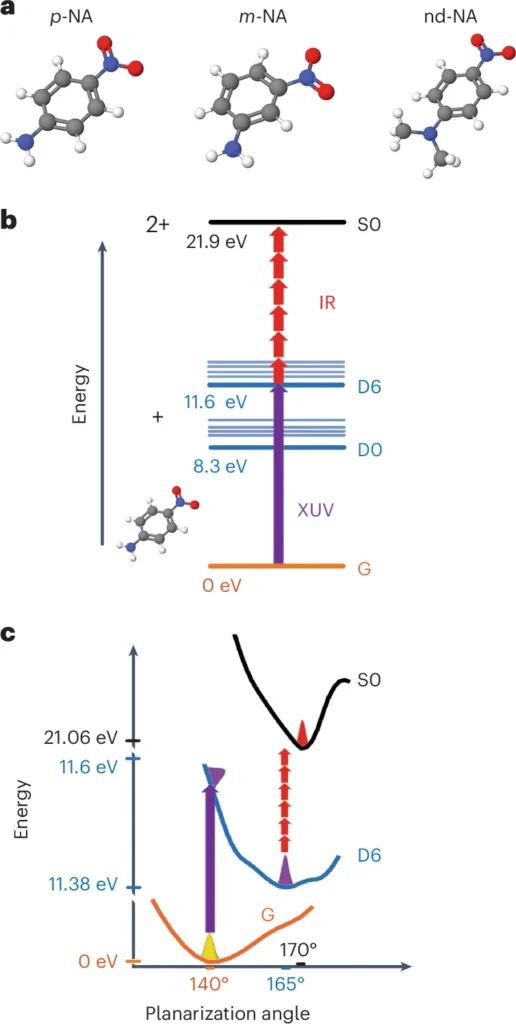
Comprehending Donor-π-Acceptor Molecules:
Central to these investigations are Donor-π-Acceptor Molecules. These molecules comprise three essential components: a donor group that supplies electrons; an π-bridge that facilitates electron transfer; and an acceptor group that accepts the electrons. The π-bridge comprises conjugated systems that enable efficient electron transport between the donor and acceptor. These molecules are excellent subjects for investigating electron transfer, especially light excitation.
These systems are particularly advantageous in organic photovoltaics and molecular electronics due to their ability to transform light energy into electrical energy with great efficiency. To optimize their function, it is essential to comprehend electron transfer kinetics from the donor to the acceptor, which is the focus of femtosecond investigations.
Photoionization in Donor-π-Acceptor Molecules:
Photoionization denotes the process whereby light ejects electrons from atoms or molecules, resulting in the formation of ions. In donor-acceptor systems, exposure to light causes the donor to absorb a photon and become excited. The excitation energy induces the electron to traverse the π-bridge towards the acceptor, hence facilitating charge separation.
Photoionization is critical because it initiates the entire electron transfer process. In its absence, the electrons would remain tethered to the donor, preventing any mobility. Scientists can trigger and examine the ensuing ultrafast electron dynamics by exposing these devices to laser light. Time-resolved measurements.
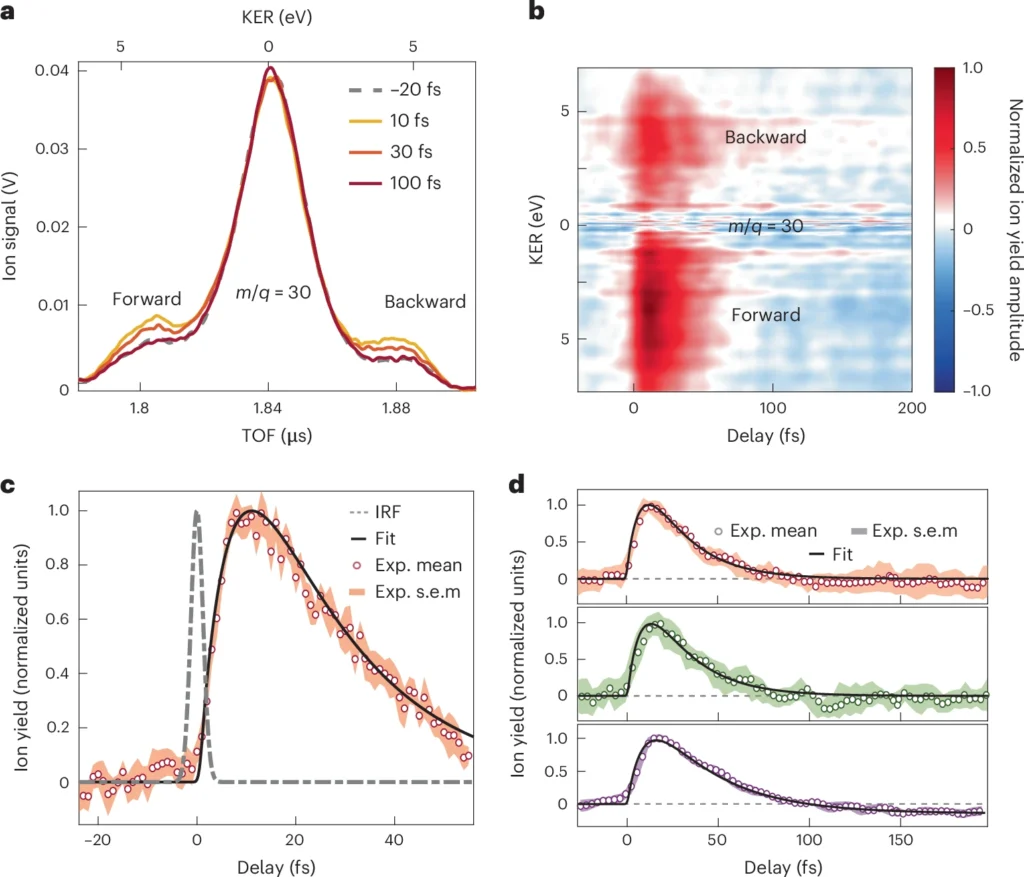
Femtosecond Timescale: An Essential Concept
A femtosecond is an exceedingly brief unit of time, equal to one quadrillionth of a second (10⁻¹⁵ seconds). To put this into perspective, if we extend one second to the lifespan of the universe, a femtosecond would still be shorter than a blink. This timescale is crucial because numerous chemical and physical events, like electron transfer, occur at exceedingly rapid rates, often too swift for conventional methods to detect.
In donor-acceptor systems, electron transfer may occur within a few femtoseconds following photoionization. By quantifying these ultrafast phenomena, researchers can document the instantaneous reactions of electrons to light, elucidating essential aspects of the progression of these processes.
Electron transfer mechanisms in Donor-π-Acceptor Molecules configurations:
The electron transfer mechanism in Donor-π-Acceptor Molecules generally entails the migration of electrons from the donor, over the π-bridge, to the acceptor. The π-bridge is essential, serving as a conduit for electron transport. Depending on the molecular architecture, this transfer may occur rapidly or slowly, with the optimal condition being a swift and efficient transfer to enhance energy conversion.
Numerous factors affect this mechanism, including the potency of the donor and acceptor, the length and composition of the π-bridge, and the surrounding environment, such as the solvent or molecule configuration. Optimizing these parameters is critical for improving these systems’ performance in real-world applications.
The Function of Ultrafast Laser Pulses:
Ultrafast lasers serve as the principal instrument for investigating femtosecond electron transport. These lasers produce exceptionally brief light pulses, often within the femtosecond range, capable of triggering photoionization in Donor-π-Acceptor Molecules. By meticulously regulating the wavelength and intensity of the laser pulses, researchers can initiate and monitor the ensuing electron dynamics with exceptional precision.
These investigations have been essential in elucidating the precise paths that electrons traverse as they migrate via the π-bridge and into the acceptor. Sub-10-fs planarization process.
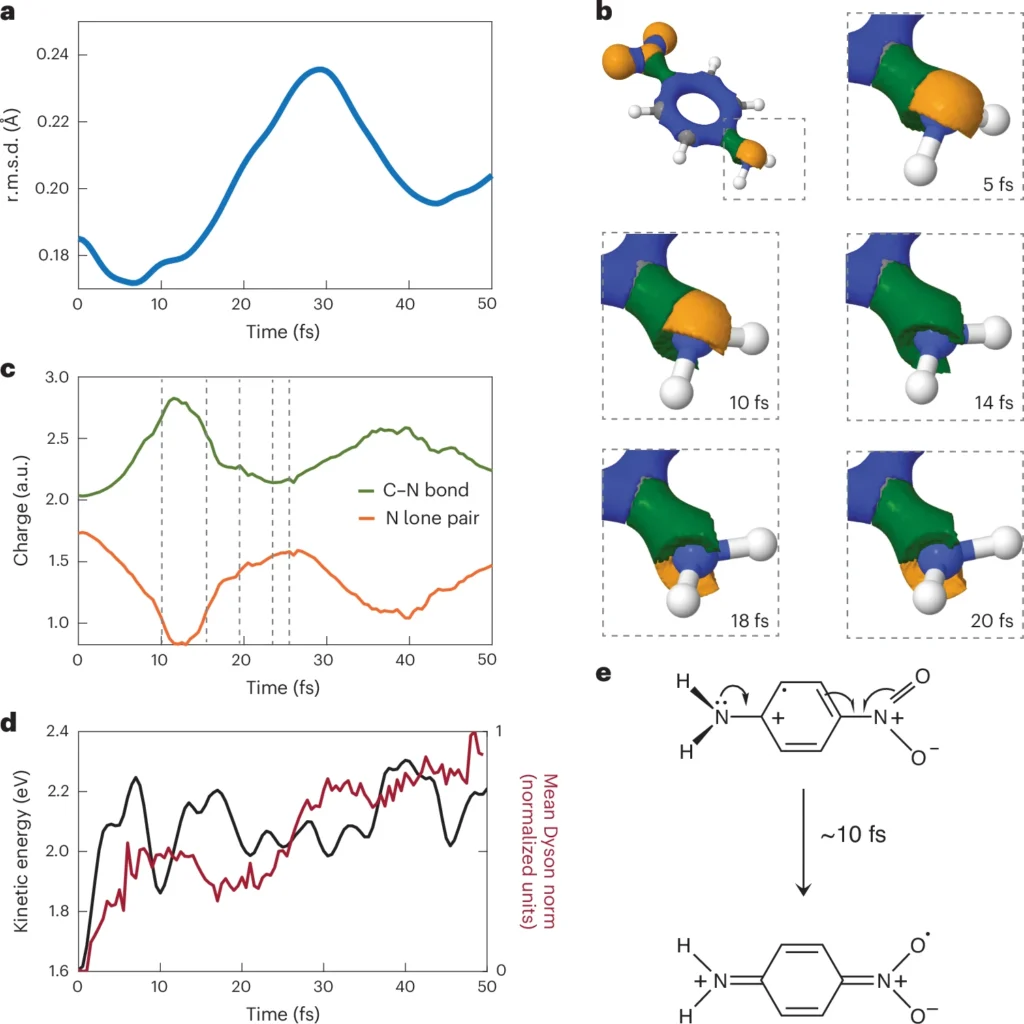
Charge separation and recombination:
The primary goal of electron transfer research is to achieve effective charge separation. Upon excitation, an electron migrates toward the acceptor, resulting in a system comprising a positive charge (the ionized donor) and a negative charge (the electron at the acceptor). Rapid recombination of these charges dissipates the energy, making it unfavorable in applications like solar cells or molecular electronics.
Researchers aim to improve the efficiency of these systems by preventing recombination through modifications to the molecular structure or by employing environmental factors that promote charge separation.
Experimental Methodologies for Monitoring Femtosecond Electron Transfer:
To study electron transfer kinetics in donor-acceptor systems, researchers use a variety of sophisticated methods:
Pump-probe spectroscopy employs two laser pulses: one to excite the system (the pump) and another to assess the response (the probe) at different time intervals.
Time-resolved photoelectron spectroscopy: Time-resolved photoelectron spectroscopy measures the speed of the electrons that are released during photoionization to help us understand how the transfer works.
Ultrafast transient absorption spectroscopy: Researchers can track electron mobility within the molecule by monitoring temporal variations in light absorption.
Determinants Influencing Electron Transfer Dynamics:
Various factors influence the electron transport kinetics of donor-acceptor compounds.
Molecular structure: The molecular structure, including the dimensions and characteristics of the donor, acceptor, and π-bridge, can facilitate or impede electron transfer.
Solvent effects: The ambient environment can either stabilize or destabilize charge-separated states.
Temperature: Elevated temperatures may enhance vibrational activity, thereby accelerating or obstructing electron transmission.
Significant Research in Femtosecond Electron Transfer:
Multiple studies have investigated the complexities of electron transfer in donor-acceptor systems. Researchers have effectively shown that altering molecular architectures can improve electron transfer rates and reduce recombination. In certain systems, the incorporation of particular functional groups into the π-bridge has demonstrated a substantial enhancement in charge separation efficiency. Sub-30-fs relaxation dynamics.
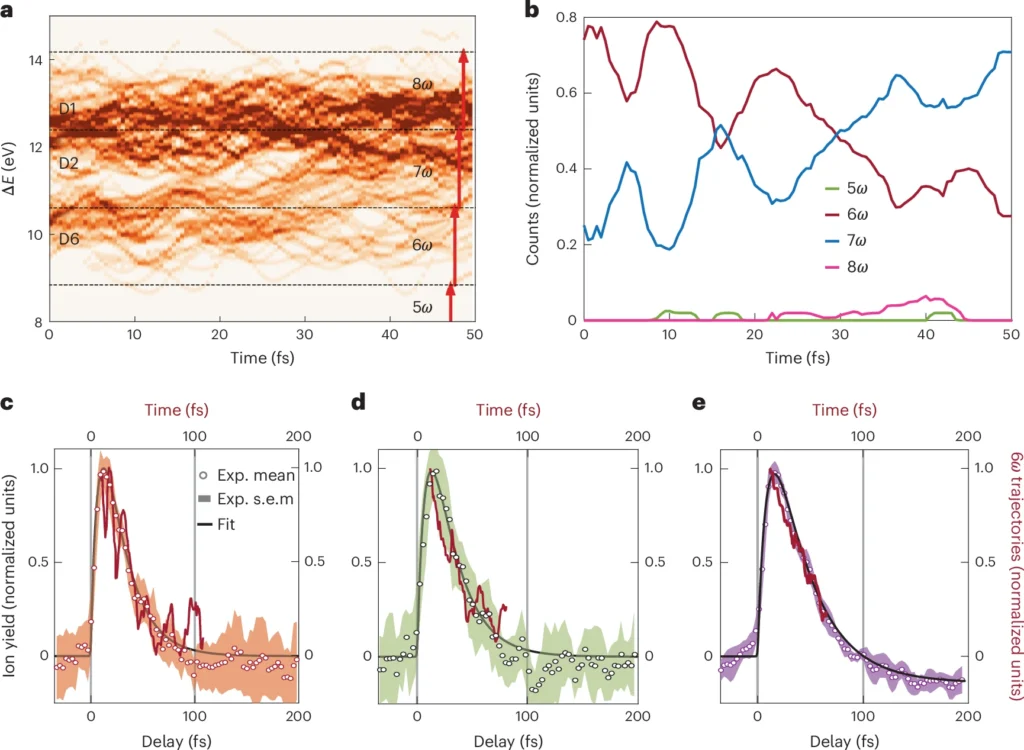
Applications of Femtosecond Electron Transfer:
Understanding femtosecond electron transport dynamics has practical implications for a variety of industries.
Solar cells: Improved electron transfer can increase the efficiency of organic photovoltaic devices in solar cells.
Molecular electronics: Accelerated electron mobility is crucial for the advancement of smaller, more efficient electronic components.
Molecular switches and sensors: Electron transport is fundamental to numerous devices that depend on molecular-level alterations for operation.
Obstacles in Investigating Femtosecond Dynamics:
Notwithstanding progress, the investigation of femtosecond electron transport continues to pose significant challenges. The principal challenge resides in recording such rapid processes, necessitating specialized apparatus and highly accurate measurements. Moreover, analyzing the data from these experiments can be intricate, frequently necessitating advanced models and simulations to comprehensively grasp the phenomena occurring at the atomic level.
Theoretical models of electron transfer in Donor-π-Acceptor Molecules:
Conventional models such as Marcus’ theory have established a robust framework for comprehending electron transfer, yet they fail to precisely characterize the ultrafast dynamics seen on the femtosecond scale. Quantum mechanical models and non-adiabatic transition theory have been used by researchers to make more accurate predictions about how electrons will behave during photoionization.
Prospective Avenues in Electron Transfer in Donor-π-Acceptor Molecules Investigation:
The domain of femtosecond electron transport is rapidly expanding. With advancements in experimental methodologies, researchers are discovering novel methods to alter electron dynamics in Donor-π-Acceptor Molecules. Future research may concentrate on developing more efficient molecular architectures and investigating practical applications in energy conversion and electronic devices.
Final Assessment:
The investigation of femtosecond electron transport kinetics in Donor-π-Acceptor Molecules is at the forefront of ultrafast research. Comprehending electron mobility inside systems, from solar cells to molecular electronics, is essential for advancing future technologies. As academics persist in investigating this domain, we might anticipate novel advances that extend the boundaries of speed and efficiency.
Frequently Asked Questions:
1). In simple terms, what is a femtosecond?
A femtosecond is one quadrillionth of a second, an exceedingly brief duration.
2). What is the significance of the π-bridge in Donor-π-Acceptor Molecules?
The π-bridge functions as a conduit, facilitating the seamless transfer of electrons between the donor and acceptor.
3). What are the prevalent applications of femtosecond electron transfer research?
Applications encompass enhancing solar cells, advancing molecular electronics, and constructing molecular switches.
4). In what manner does temperature influence electron transfer inside these systems?
Elevated temperatures may enhance molecular vibrations, thereby accelerating or obstructing electron transmission.
5). What is the predominant method for assessing femtosecond dynamics?
We extensively employ pump-probe spectroscopy to investigate femtosecond electron transport kinetics.
For more chemistry blogs, visit chemistry Master