Table of Contents
Introduction of Asymmetric Organocatalysis:
An extremely useful tool in synthetic chemistry is asymmetric organocatalysis, which makes it possible to make chiral compounds very quickly and selectively. It has transformed the domain of organic synthesis and shows great potential for diverse industries, such as medicines, agrochemicals, and materials research.
Definition and Significance of Asymmetric Organocatalysis:
Asymmetric organocatalysis uses small organic compounds as catalysts to make asymmetric processes possible. This lets precise control over stereochemistry happen when chiral centers are formed. This technology’s significance in contemporary organic synthesis lies in its ability to create intricate compounds with precise stereochemistry. Asymmetric organocatalysis is important because it allows for the production of enantiomerically pure molecules, which are crucial in drug discovery, asymmetric catalysis, and materials science.
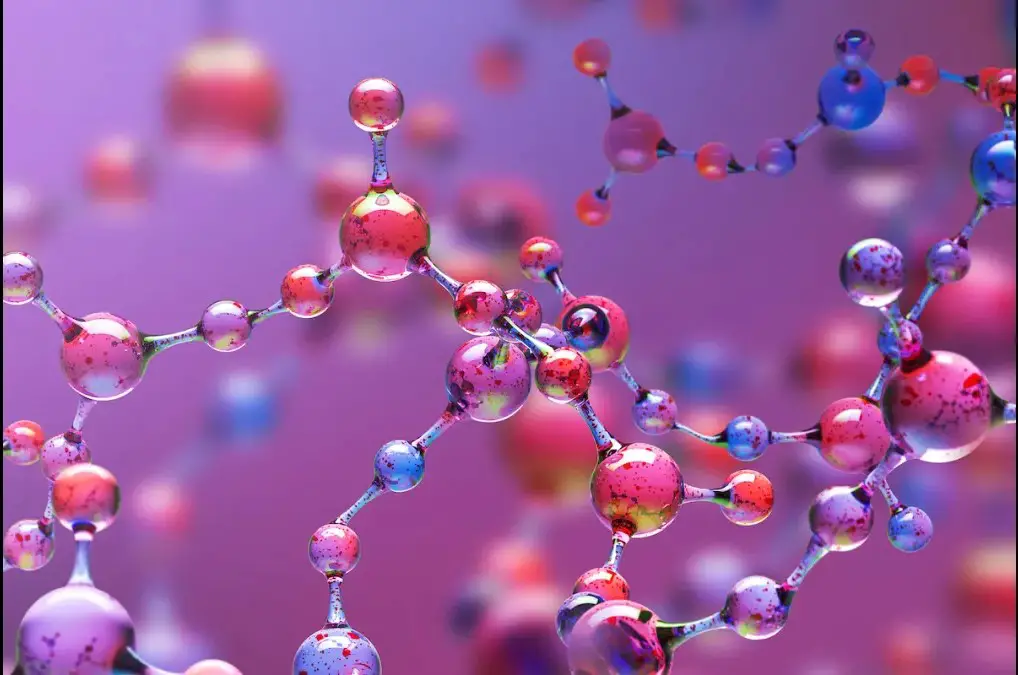
Historical Background of Asymmetric Organocatalysis:
Scientists began investigating techniques to introduce chirality into organic compounds in the mid-20th century, marking the origins of asymmetric catalysis. Nobel laureates Ryoji Noyori and William S. Knowles’ groundbreaking work in asymmetric hydrogenation laid the groundwork for the advancement of asymmetric organocatalysis. During the beginning of the 21st century, the groundbreaking contributions of David W. C. MacMillan and Benjamin List sparked a renewed fascination with organocatalytic techniques, resulting in significant progress in the field and broadening its range of applications.
Mechanism of Asymmetric Organocatalysis:
The mechanism of asymmetric organocatalysis involves the utilization of chiral, tiny organic molecules, referred to as organocatalysts, to enable enantioselective reactions. The process usually has several catalytic cycles where the organocatalyst reacts with the substrate to make reactive intermediates that have clear stereochemistry. Non-covalent interactions between the organocatalyst and the substrate, such as hydrogen bonding, electrostatic interactions, and stacking, achieve chiral induction. These interactions result in preferential chiral product synthesis. The catalytic cycle involves several crucial processes, including the activation of the substrate, the production of stereoselective bonds, and the regeneration of the catalyst. These steps are essential for maintaining the sustainability of the catalytic process.
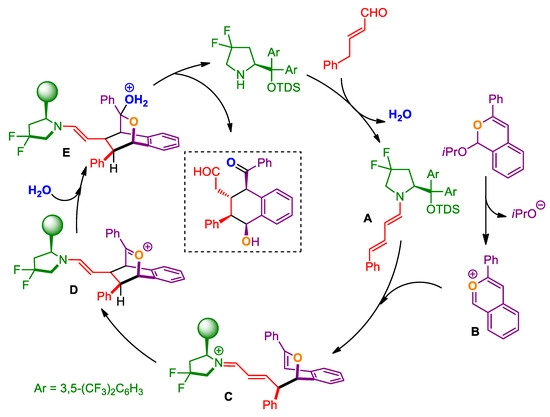
Explanation of Chiral Catalysts:
In asymmetric organocatalysis, chiral catalysts are very important because they give reaction intermediates chirality, which controls the stereochemical outcome of the reaction. These catalysts are usually tiny organic compounds that have one or more asymmetric centers. This allows them to differentiate between enantiomers and facilitates the production of single enantiomers with a high level of selectivity. We can classify chiral catalysts into several categories, including chiral amines, chiral phosphines, chiral thioureas, and chiral Brnsted acids. Each of these groups has distinct benefits in terms of catalytic activity and compatibility with different substrates.
Key Steps in the Catalytic Cycle:
The catalytic cycle of asymmetric organocatalysis consists of a series of critical phases, beginning with the chiral catalyst activating the substrate. The subsequent development of reactive intermediates allows for the selective creation of chemical bonds with a specific spatial arrangement, resulting in the production of chiral compounds. The inherent chirality of the organocatalyst and its interactions with the substrate determine the stereochemical result of the reaction. The catalyst regenerates after forming the product, completing the catalytic cycle and enabling its reuse in future reactions.
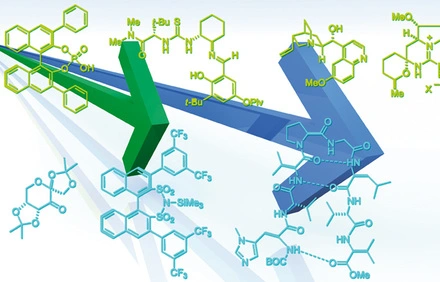
Recent Advances:
Researchers’ persistent endeavors to create efficient and selective catalytic systems have led to notable improvements in asymmetric organocatalysis in recent years. Researchers have developed novel catalyst designs, like bifunctional and multifunctional organocatalysts, to overcome the challenges of conventional catalysts, offering enhanced reactivity and a broader range of substrates. Furthermore, advancements in computational chemistry and high-throughput screening techniques have expedited the rapid identification and enhancement of organocatalysts, hastening the development of novel approaches to asymmetric synthesis.
Novel Catalyst Designs:
Novel catalyst designs have broadened the range of tools that chemists can use to create complicated compounds with exceptional efficiency and accuracy. Bifunctional organocatalysts, which are molecules with multiple catalytic motifs, have gotten a lot of attention because they can speed up complex reactions while being very selective about the stereochemistry involved. Also, catalysts with both covalent and non-covalent interactions are very good at speeding up asymmetric reactions like making C-C bonds, adding conjugates asymmetrically, and changing carbonyl compounds selectively based on their enantioselectivity. These new catalysts utilize various catalytic capabilities to create versatile and sustainable options for synthesizing complex molecules in both academic and industrial settings.
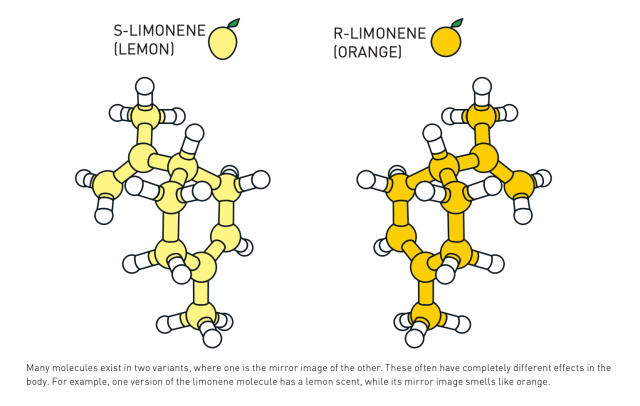
Substrate Scope Expansion:
Substrate scope extension, along with catalyst development, is a significant area of research in asymmetric organocatalysis. Its objective is to widen the variety of chiral building blocks and natural products that can be obtained. The development of substrate-controlled reactions and transition metal-catalyzed cross-coupling techniques has enabled the creation of a wide range of molecular frameworks with precise stereochemical accuracy. Using bioorthogonal chemistry, photoredox catalysis, and asymmetric organocatalysis together has also opened up new ways to make complex molecular structures with mild reaction conditions. These advancements have significant potential for use in drug discovery, materials science, and chemical biology. In these fields, it is crucial to have access to a wide range of compounds that are both varied and stereochemically pure. This is necessary for conducting investigations on the relationship between structure and activity as well as for designing functional materials.
Applications of Asymmetric Organocatalysis:
Asymmetric organocatalysis is widely used in industries such as pharmaceuticals, agrochemicals, and fine chemical manufacturing. People value it for its ability to produce enantiomerically pure molecules with great efficiency and selectivity. In the pharmaceutical industry, asymmetric organocatalysis is critical for synthesizing chiral drug candidates and pharmaceutical intermediates. It allows for fine control over stereochemistry, which is critical for ensuring biological activity and pharmacokinetic characteristics. Therefore, in the field of agrochemicals and crop protection, asymmetric organocatalysis makes it possible to make chiral herbicides and pesticides that work better and have less of an impact on the environment. Furthermore, in the field of fine chemical manufacturing, asymmetric organocatalysis provides environmentally friendly and economically efficient methods for producing chiral building blocks. These building blocks are essential for creating flavors, perfumes, and specialty chemicals, which are in high demand for various industrial applications and consumer needs.
Drug Synthesis:
Production of chiral medicines is a significant application of asymmetric organocatalysis, where precise control over stereochemistry is critical for therapeutic effectiveness and safety. Asymmetric organocatalytic methods have been used to synthesize many pharmaceuticals, including small-molecule medications, natural products, and biologics. People have used these methods to treat cardiovascular illnesses, cancer, infectious diseases, and neurological disorders. Some examples are making β-blockers, anti-inflammatory drugs, antiviral drugs, and chiral compounds that are used as building blocks for making peptide and nucleoside analogs. These examples emphasize the adaptability and usefulness of asymmetric organocatalysis in the process of discovering and developing pharmaceuticals.
Fine Chemical Manufacturing:
Asymmetric organocatalysis is a useful technique in fine chemical manufacturing for creating chiral building blocks and intermediates. These are important in producing specialized chemicals, tastes, and fragrances. Getting enantiomerically pure substances is important for making functional materials and high-value goods because it makes it easy to build complex molecular structures with precise stereochemical control. We have used asymmetric organocatalytic methods to produce chiral alcohols, amines, esters, and lactones. These compounds are important for creating medicines, agrochemicals, and performance materials. Furthermore, the ability to scale up and maintain asymmetric organocatalysis over time makes it a highly appealing choice for industrial use. It provides environmentally friendly and economically advantageous alternatives to conventional synthetic methods.
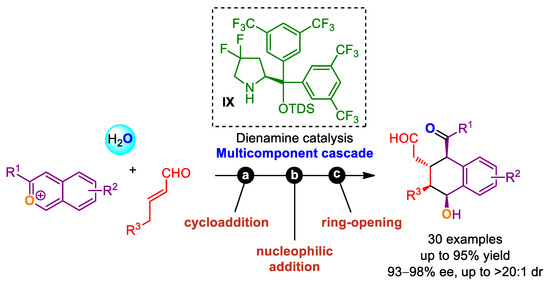
Challenges and Future Directions:
Although there has been notable advancement in the field of asymmetric organocatalysis, there are still several problems and prospects for future research. A key obstacle is the creation of durable and environmentally friendly catalytic systems that can facilitate a diverse array of asymmetric reactions with exceptional effectiveness and specificity. Existing techniques may have limitations in terms of the range of substrates they can work with, the stability of the catalyst used, and the capacity to scale up the reaction. These constraints hamper their broad use in industrial settings. To tackle these issues, it is necessary to employ interdisciplinary methods that integrate synthetic chemistry, computational modeling, and process engineering. This will enable the creation of customized catalysts and the optimization of reaction conditions for real-world uses. Furthermore, the development of innovative catalytic techniques and reaction mechanisms has the potential to broaden the synthetic capabilities of asymmetric organocatalysis and explore uncharted chemical territory. Current research topics include the use of artificial intelligence and machine learning methods to improve catalyst design and reaction optimization.
Limitations of Current Methodologies:
Despite its numerous advantages, the current methodologies for asymmetric organocatalysis have several constraints that impede its extensive use in commercial settings. One problem is that present catalysts can only react with a small number of substances. They may also react less strongly with certain functional groups or classes of chemicals. Furthermore, addressing the capacity of organocatalysts to maintain stability under specific reaction circumstances and their compatibility with various reaction media is crucial, particularly in optimizing large-scale synthesis processes. Moreover, the scalability of asymmetric organocatalytic techniques presents difficulties because they depend on using precise amounts of catalysts and result in the production of chemical waste. To overcome these constraints, it is necessary to create novel catalytic systems that exhibit better reactivity, selectivity, and recyclability. Additionally, optimizing reaction conditions is crucial to improving efficiency and sustainability.
Emerging Trends in Research:
The current focus of asymmetric organocatalysis research is on identifying and overcoming existing limits, as well as investigating novel catalytic techniques for the synthesis of complex molecules. A new trend in the field is making cooperative catalytic systems that use the actions of many catalytic motifs together to make difficult conversions easier while still being very selective and efficient. Cooperative catalysis refers to the process of activating numerous substrates simultaneously using different catalysts. This leads to cascade reactions and the quick formation of intricate molecular structures. A growing approach is combining bioorthogonal chemistry and organocatalysis to create bioactive compounds and useful materials with gentle reaction conditions. It is possible to change biomolecules in complex biological settings using bioorthogonal reactions. This opens up new opportunities for drug development and chemical biology uses.
Conclusion:
Asymmetric organocatalysis is a highly effective method for synthesizing chiral compounds with great efficiency and selectivity. It provides flexible methods for synthesizing complicated chemicals in many sectors. Asymmetric organocatalysis is crucial in various fields, such as drug discovery and fine chemical manufacturing. It enables the production of enantiomerically pure molecules with specific properties and functions, leading to breakthroughs in pharmaceuticals, agrochemicals, and materials science. Recent advancements in catalyst design, broadening substrate scope, and developing reaction techniques have increased the range of synthetic tools available to chemists. This allows for the rapid creation of intricate molecular structures under gentle reaction conditions. Nevertheless, there are still obstacles to overcome in the form of limited material availability, the catalyst’s stability, and the ability to scale up the reaction. This emphasizes the importance of continuous research and development.
Frequently Asked Questions:
1). What is organocatalysis?
In organocatalysis, organic compounds act as catalysts to speed up chemical reactions. Organocatalysis is a chemical reaction in which organic molecules act as catalysts. These catalysts promote the formation or breaking of chemical bonds without relying on metal-containing catalysts.
2). What is the significance of asymmetric organocatalysis?
Asymmetric organocatalysis makes it possible to make chiral compounds precisely. These compounds are important in materials research, medicines, and agrochemicals because they have special biological and physical properties.
3). Can you provide some instances of asymmetric organocatalysts?
Asymmetric organocatalysts commonly used are chiral amines, thioureas, and phosphines. These catalysts can create chirality in reaction intermediates and facilitate enantioselective transformations.
4). What are the differences between asymmetric organocatalysis and other asymmetric synthesis methods?
Asymmetric organocatalysis has benefits such as gentle reaction conditions, little toxicity, and environmental friendliness, in contrast to conventional approaches that rely on transition metal catalysts or enzyme catalysis.
5). What are the potential outcomes or possibilities for the future development of asymmetric organocatalysis?
Future research in asymmetric organocatalysis aims to surmount current limitations, including the scope of substances that can serve as substrates and the ability to recycle catalysts. Additionally, it wants to investigate new catalytic systems and reaction techniques to expand its usefulness in synthetic chemistry.
For more chemistry blogs, visit chemistry Master