Table of Contents
Overview of Symmetry-breaking of Carbon Dioxide Dimer:
Symmetry is important in molecular chemistry because it greatly influences molecules’ characteristics and actions. Symmetry governs how a molecule interacts with light, other molecules, and its components. However, what happens when we disrupt this symmetry? Symmetry-breaking, a phenomenon that occurs in complex systems like the carbon dioxide dimer, can produce various fascinating results. This article looks at the complicated dynamics of breaking symmetry in photoionized Carbon dioxide dimers. It looks at the basic ideas, the results of experiments, and the bigger effects of these events.
Principles of Carbon Dioxide Dimers:
One carbon atom double-bonded to two oxygen atoms forms the chemical known as carbon dioxide. The Earth’s atmosphere is widely recognised for its important function. When two molecules of carbon dioxide combine, they create a dimer, which is a compound with unique characteristics. The primary stabilisation of the Carbon dioxide dimer is attributed to weak van der Waals forces, which are a sort of intermolecular contact. Despite their weakness, these forces play a critical role in numerous atmospheric and chemical processes.
The structure of the Carbon dioxide dimer is made up of two molecules that are close to each other but not chemically bound to each other. In this way, their properties are affected by each other. This interaction is especially intriguing in the field of atmospheric chemistry, as Carbon dioxide dimers have the potential to influence the Earth’s radiative balance and the processes involved in cloud formation. Gaining a comprehensive understanding of the basic characteristics of these dimers, such as their creation, durability, and interactions, is essential for more extensive environmental and chemical investigations. The electronic structure of the ionized carbon dioxide dimer at the Franck-Condon geometry.
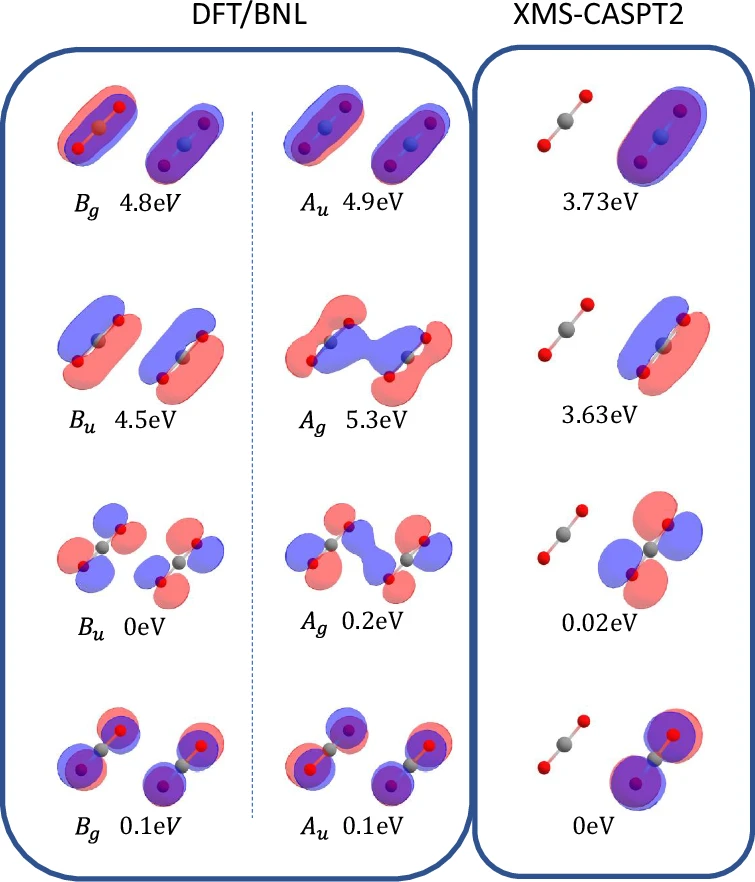
Photoionization: Fundamental Concepts and Mechanisms
Photoionization is the phenomenon in which a photon, which is a unit of light, transfers sufficient energy to a molecule, causing the expulsion of one or more electrons and the creation of a positively charged ion. This method is essential for comprehending molecular dynamics and is also a crucial instrument for investigating the electronic structure of molecules.
Photoionization has the potential to greatly modify the electrical and structural characteristics of Carbon dioxide dimers. Photon absorption can cause electronic excitations, altering the electron distribution in the molecule and potentially resulting in the disruption of the weak van der Waals contacts that maintain the dimer’s cohesion. Photoionization is a highly effective technique for investigating the complex aspects of symmetry-breaking dynamics.
Molecular chemistry involves the study of symmetry:
Molecular symmetry pertains to the harmonious organisation of atoms and electrons inside the molecular framework. The balance of symmetry in a molecule can manifest in three ways: rotational symmetry, where the molecule remains unchanged after rotation around an axis; reflectional symmetry, where the molecule can be mirrored along a plane; and translational symmetry, where repeating units of the molecule are evenly spaced.
The significance of symmetry is crucial in determining the interaction of Carbon dioxide dimers with light and other molecules. The molecular symmetry can have a significant impact on the spectroscopic characteristics, reactivity, and stability of a molecule. For example, the orderly positioning of atoms in a molecule can result in distinct vibrating patterns that can be identified by infrared spectroscopy. Comprehending these symmetries is essential for interpreting empirical evidence and forecasting molecular behaviour. Time-resolved snapshots of the CASPT2 dynamics on the ground and excited states of the {(C{O}_{2})}_{2}^{+} dimer ion. The Mulliken charge on each monomer is indicated.
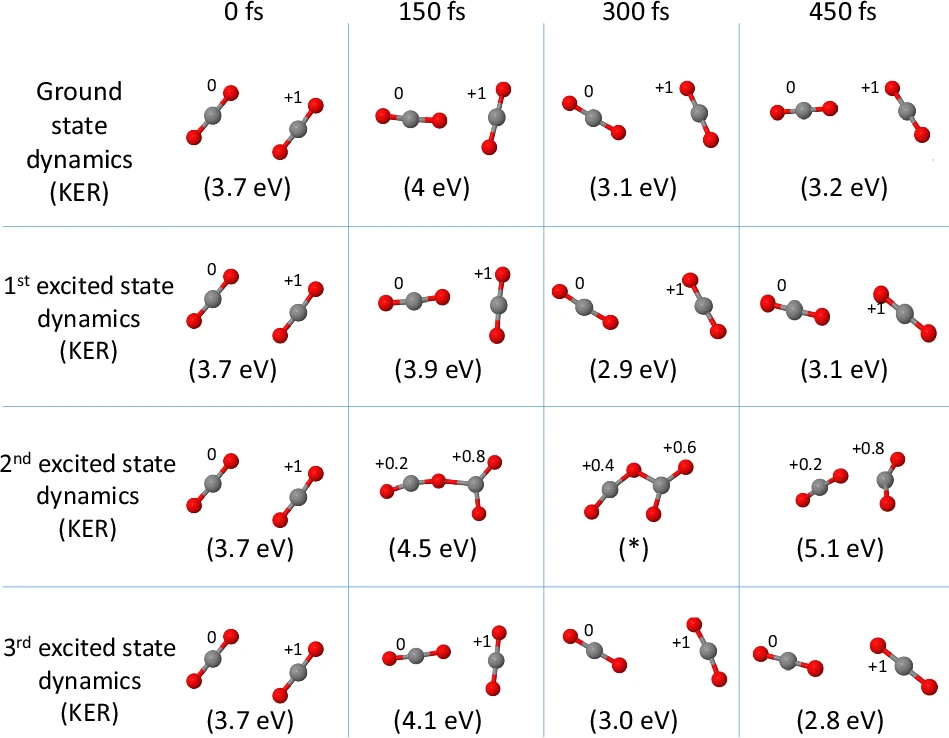
An Explanation of Symmetry-Breaking:
Symmetry-breaking is the phenomenon that arises when an external force or factor disturbs the symmetrical configuration of atoms or electrons within a molecule. These occurrences might arise spontaneously, for example, due to thermal fluctuations, or be triggered by external influences such as photoionization or chemical processes. When symmetry is disturbed, the characteristics of the molecule can vary substantially, leading to unexpected behaviours and reactions.
In the case of Carbon dioxide dimers, symmetry-breaking can lead to changes in the vibrational and rotational states of the molecules. These modifications can impact the stability of the dimer and its interactions with other molecules. For instance, the process of symmetry-breaking can enhance the reactivity of a molecule or alter its absorption spectrum, thereby enabling its detection using various spectroscopic techniques. Gaining a comprehensive understanding of the mechanisms that cause symmetry breaking is crucial for accurately predicting these alterations and their consequences. Time-resolved KER distribution in Coulomb explosion of CO2 dimers.
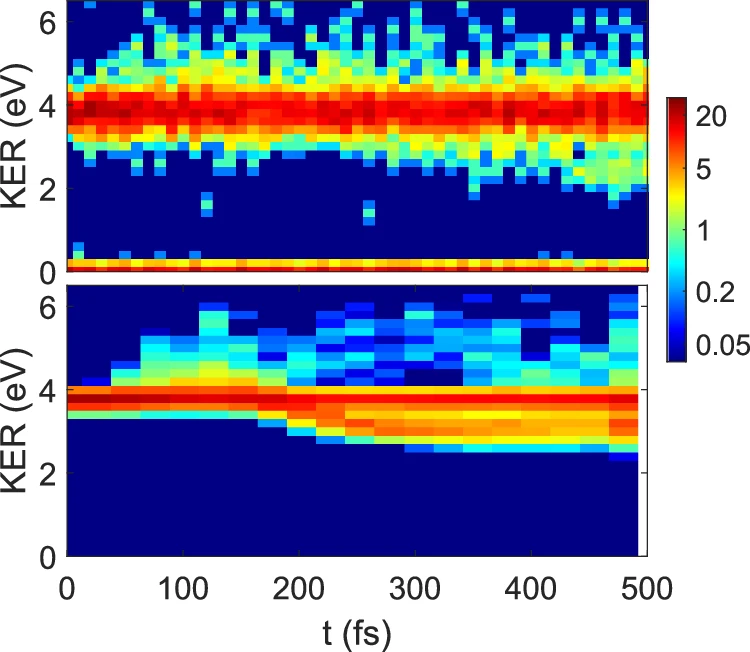
Methods for Observing Symmetry-Breaking in Experiments:
Advanced experimental techniques are necessary to observe symmetry-breaking in real-time. Photoelectron spectroscopy is a frequently employed technique that entails the measurement of electron energy released from a molecule during photoionization. This method offers comprehensive insights into the electronic configuration of the molecule and its alterations during the process of symmetry disruption.
Ultrafast laser spectroscopy is a potent method that employs very brief laser pulses to observe rapid molecular processes. This technique is especially valuable for investigating the kinetics of symmetry-breaking, as it can offer instantaneous data on the alterations taking place within the molecule. Nevertheless, conducting these experiments can be arduous because of the requirement for meticulous management of the experimental parameters and the analysis of intricate data.
Theoretical models and computational approaches:
Theoretical models and computational simulations are essential in investigating symmetry-breaking dynamics, as they provide valuable insights that complement experimental results. Quantum mechanical models, such as ab initio approaches, offer a comprehensive depiction of the electrical configuration of molecules. These models can forecast the behaviour of electrons in a molecule under different circumstances, especially during the process of photoionization.
Molecular dynamics simulations, in contrast, are employed to investigate the temporal movement of atoms and molecules. These simulations can offer a valuable understanding of the development of symmetry-breaking and the variables that impact this phenomenon. Through the process of comparing theoretical predictions with actual evidence, scientists can enhance their models and advance their comprehension of the fundamental systems involved.
Symmetry-breaking in Carbon dioxide dimers caused by photoionization:
When carbon dioxide dimers are exposed to photoionization, several intriguing phenomena can take place. Empirical investigations have demonstrated that the photoionization process can result in the creation of profoundly stimulated electronic states in the dimer. These states frequently lead to the dissociation of the dimer into individual CO2 molecules or the reformation of the dimer in an alternative arrangement.
The result of photoionization is influenced by various parameters, such as the energy of the photon that is absorbed and the initial electronic and vibrational state of the dimer. For example, photons with high energy can supply sufficient energy to both ionise the dimer and elevate it to higher electronic states, resulting in more substantial alterations in its structure. An in-depth analysis of these processes facilitates comprehension of the intricacy of symmetry-breaking dynamics in such systems.
Electronic Structure and Vibrational Dynamics:
Study of the arrangement of electrons in atoms and molecules and the movement of atoms in a vibrating system.
Photoionization significantly alters the electrical structure of a molecule. Removing an electron from CO2 dimers can cause a redistribution of electronic density, which subsequently impacts the bond lengths and angles in the molecule. This redistribution can induce the dimer to assume novel shapes or even disassemble into distinct CO2 molecules.
Furthermore, the interplay between electronic states and vibrational modes is of utmost importance in the dynamics of symmetry-breaking. Electronic stimulation can cause the electronic and vibrational states to become linked, causing energy to move from the electronic mode to the vibrational mode. This process might disintegrate the dimer or the creation of additional vibrational states, hence adding complexity to the system’s dynamics.
Effects of Symmetry Disruption on Molecular Stability:
The phenomenon of symmetry-breaking can exert a significant influence on the stability of molecular systems. When symmetry is disrupted in Carbon dioxide dimers, it frequently alters the energy distribution within the molecule, impacting its stability and reactivity. For example, the creation of highly stimulated electronic states can increase the likelihood of the dimer breaking apart, resulting in the release of CO2 molecules.
The shifts in stability are significant not only for comprehending the fundamental characteristics of Carbon dioxide dimers but also for their wider impact on atmospheric chemistry. As an illustration, the separation of Carbon dioxide dimers in the higher regions of the atmosphere might contribute to the creation of unattached atoms that have a significant impact on atmospheric phenomena such as the reduction of ozone and the greenhouse effect.
Comparative analysis with other molecular systems:
Symmetry-breaking is not exclusive to Carbon dioxide dimers; it is a prevalent occurrence in numerous molecular systems. For example, analogous mechanisms have been documented in other simple molecules such as O2, N2, and H2O. By comparing the behaviour of Carbon dioxide dimers with other systems, useful insights into the general principles of symmetry-breaking dynamics can be gained.
For example, in O2 dimers, symmetry-breaking can lead to the creation of reactive oxygen species, which are crucial in both biological and atmospheric processes. In N2 dimers, symmetry-breaking can change the molecule’s absorption spectra, making it a subject of interest in studies connected to planetary atmospheres. By examining these and other systems, scientists can acquire a more thorough knowledge of the processes that impact symmetry-breaking and its repercussions.
Implications for Atmospheric Chemistry and Environmental Science:
The study of Carbon dioxide dimers and their symmetry-breaking behaviour has substantial implications for atmospheric chemistry. CO2 is a key greenhouse gas, and understanding its behaviour in multiple forms, including dimers, is vital for accurate climate prediction. Symmetry-breaking in Carbon dioxide dimers can influence how these molecules interact with radiation, influencing the Earth’s energy balance.
Moreover, the results of symmetry-breaking, such as free CO2 molecules or other reactive species, can engage in different atmospheric processes. For instance, the creation of Carbon dioxide dimers and their subsequent dissociation can change the concentration of greenhouse gases in the atmosphere, causing global warming. Understanding these processes is vital for establishing successful methods for reducing climate change. The onset time of the high and low KER events as a function of the EUV-pump EUV-probe delay
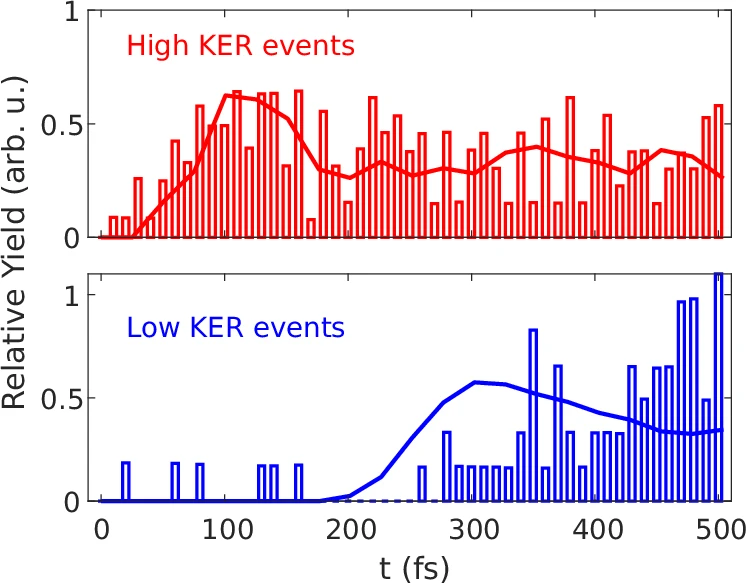
Technological and Practical Applications:
Beyond the academic interest, the study of symmetry-breaking dynamics in Carbon dioxide dimers has practical implications in numerous domains. In materials research, understanding these processes can lead to the development of novel materials with specialised optical or electrical properties. For example, materials that exhibit regulated symmetry-breaking could be employed in sensors or optical systems.
In environmental monitoring, insights from these studies could improve our understanding of pollutant behaviour and atmospheric chemistry. For example, observing the creation and separation of Carbon dioxide dimers could yield important data regarding the levels of CO2 and other greenhouse gases in the atmosphere. This data is essential for the development of precise climate models and the anticipation of environmental fluctuations.
Potential Areas for Future Investigation and Research:
There is great potential for additional investigation on the topic of symmetry-breaking dynamics. Novel experimental approaches, such as X-ray free-electron lasers, provide fresh opportunities for investigating these processes with unparalleled precision. These approaches can yield real-time data on the kinetics of symmetry-breaking, providing novel insights into the behaviour of molecular systems.
Furthermore, there is the possibility of extending studies to more intricate molecular systems. Although Carbon dioxide dimers are rather uncomplicated, investigating more intricate molecules or clusters could uncover novel facets of symmetry-breaking dynamics. Interdisciplinary collaborations, which involve the integration of knowledge and skills from other fields such as chemistry, physics, and environmental research, will play a pivotal role in enhancing our comprehension of these processes and their wider consequences.
In conclusion:
Examining the kinetics of symmetry-breaking in photoionized carbon dioxide dimers provides an intriguing insight into the intricate nature of molecular interactions. These processes not only enhance our comprehension of fundamental chemical concepts but also have wider ramifications for atmospheric chemistry, environmental science, and technology. As more advancements are made in study in this field, we may anticipate the discovery of other fascinating characteristics of molecular behaviour. This will enhance our comprehension of the natural world and provide valuable insights for practical applications.
Frequently Asked Questions:
1). What are the real-world consequences of investigating carbon dioxide dimers?
Examining Carbon dioxide dimers can yield significant knowledge about atmospheric chemistry, enabling us to comprehend the actions of greenhouse gases and their influence on climate change. Additionally, it can provide valuable insights for the advancement of novel materials and technologies.
2). What is the impact of symmetry on molecular interactions?
The presence of symmetry in molecules has a direct impact on their physical characteristics, including their interactions with light and other molecules. It can impact the chemical reactivity, stability, and the absorption and emission of radiation by molecules.
3). What obstacles do scientists encounter when studying symmetry breaking?
The study of symmetry-breaking presents various challenges, including the requirement for meticulous control over experimental parameters, the intricacy of interpreting intricate data, and the constraints imposed by current experimental and theoretical techniques.
4). Can these studies contribute to the resolution of environmental issues?
Indeed, comprehending the dynamics of symmetry-breaking can enhance our ability to forecast the actions of greenhouse gases and other contaminants in the atmosphere. This knowledge is vital for formulating effective approaches to alleviate climate change and environmental deterioration.
5). What are the anticipated future advancements in this field?
Possible future developments may encompass novel experimental methodologies for observing real-time symmetry-breaking phenomena, extended investigations into intricate molecular systems, and interdisciplinary partnerships that unite diverse scientific disciplines to tackle broader inquiries in the realms of chemistry and environmental science.
For more chemistry blogs, visit chemistry Master