Table of Contents
Overview:
The unwavering quest for efficient cancer treatments has led to the investigation of innovative therapeutics that can specifically target and eradicate cancer cells while minimizing harm to adjacent healthy tissue. Photodynamic therapy (PDT) and photothermal therapy (PTT) are notable among the many therapeutic techniques due to their high precision and minimal invasiveness. These therapies rely on light to activate certain chemicals and cause cytotoxic effects in cancer cells.
To improve the effectiveness of PDT and PTT, it is important to focus on targeting mitochondria, which are responsible for generating energy in cells and have a significant impact on cell survival and death. Additionally, cyanine dyes have become an important tool in this context because they can specifically gather in mitochondria and have unique photophysical properties. This article explores the intriguing field of mitochondria-targeting photodynamic and photothermal therapy, specifically examining the role of cyanine dyes. It investigates their mechanisms, uses, and potential future applications in cancer treatment.
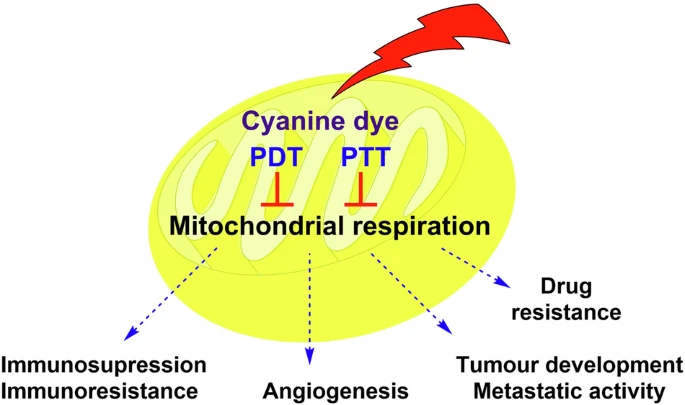
Overview of Photodynamic and Photothermal Therapy:
Definition and fundamental principles:
Photodynamic therapy (PDT) and photothermal therapy (PTT) are advanced treatment methods that utilize light-activated substances to produce therapeutic effects in certain cells, particularly cancer cells. Photodynamic therapy (PDT) involves administering a photosensitizer, a substance that becomes active when exposed to light of a specific wavelength. Activation of the photosensitizer transfers energy to the nearby molecular oxygen, leading to the production of reactive oxygen species (ROS). These reactive oxygen species (ROS) possess a high reactivity and can induce significant harm to cellular structures, resulting in either apoptosis (planned cell death) or necrosis (uncontrolled cell death).
PTT, in contrast, utilizes photothermal agents that collect light and transform it into thermal energy. The localized heat rise can eliminate cancer cells by altering the structure of proteins, disturbing cellular membranes, and causing various types of thermal harm. Another great thing about both PDT and PTT is that they don’t hurt the body. This means that they can target specific tissues by using light to target the photosensitizer or photothermal agent.
Significance in Cancer Therapy:
Historically, cancer treatment has been primarily focused on employing techniques such as surgical intervention, chemotherapy, and radiation therapy. Although these methods have shown various levels of effectiveness, they frequently result in considerable side effects due to their non-discriminatory nature, impacting both malignant and healthy tissues. Utilizing PDT and PTT can provide a more focused strategy, which has the potential to decrease the occurrence of these adverse effects. These treatments’ efficacy stems from their reliance on the targeted activation of therapeutic chemicals using light, which precisely targets the tumor location, thereby preventing harm to healthy tissues.
Furthermore, photodynamic therapy (PDT) and photothermal therapy (PTT) are especially beneficial in the treatment of cancers that exhibit resistance to traditional therapeutic approaches. PDT efficiently treats hypoxic cancers, which have low oxygen levels and are resistant to radiation and chemotherapy, by generating reactive oxygen species (ROS) in a way that is not highly dependent on oxygen concentration. PTT can effectively target and eliminate tumors that are challenging to surgically remove or unreachable using traditional procedures. Influence of mitochondrial respiration on carcinogenesis.
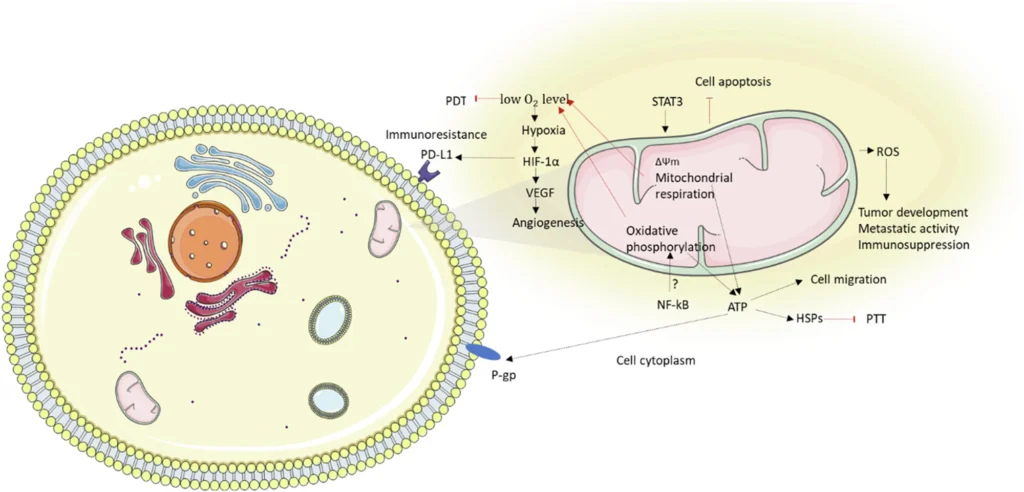
Role of Mitochondria in Cell Biology:
The role of mitochondria in cells:
Mitochondria are commonly known as the cell’s “powerhouses” because their main function is to generate adenosine triphosphate (ATP), which serves as the cell’s energy currency, through oxidative phosphorylation. Nevertheless, mitochondria serve a purpose that extends beyond their role as basic energy generators. They participate in several cellular activities, such as controlling cellular metabolism, maintaining calcium balance, and producing ROS, which serve as crucial signaling molecules in different cellular pathways.
Apoptosis regulation is one of the key functions of mitochondria. The body uses apoptosis, a tightly regulated mechanism, to eliminate damaged or no longer-needed cells. Mitochondria play a role in apoptosis by releasing pro-apoptotic substances, such as cytochrome c, into the cytoplasm in response to specific signals. The release of this substance initiates a series of processes that ultimately result in the activation of caspases, which are the enzymes responsible for carrying out the cell death process.
Targeting mitochondria for therapeutic purposes:
Due to their crucial involvement in cellular survival and apoptosis, mitochondria have emerged as an appealing focus for cancer treatment. Cancer cells frequently display modified mitochondrial activity, which facilitates their rapid proliferation and ability to evade apoptosis. For instance, cancer cells typically have an elevated mitochondrial membrane potential, which is associated with their heightened energy requirements and ability to survive apoptosis. Therapies can exploit the weaknesses of cancer cells by specifically targeting mitochondria, leading to their demise.
Mitochondria-targeting techniques aim to interfere with mitochondria’s regular operation, resulting in the initiation of apoptotic pathways and the ultimate death of cancer cells. This method can be highly efficacious in surmounting the resistance mechanisms that cancer cells build against alternative treatments, such as chemotherapy. Furthermore, by specifically targeting mitochondria, we can induce cell death in a manner unaffected by the usual mechanisms cancer cells commonly use to evade treatment. Jablonski’s diagram for the PDT and PTT.
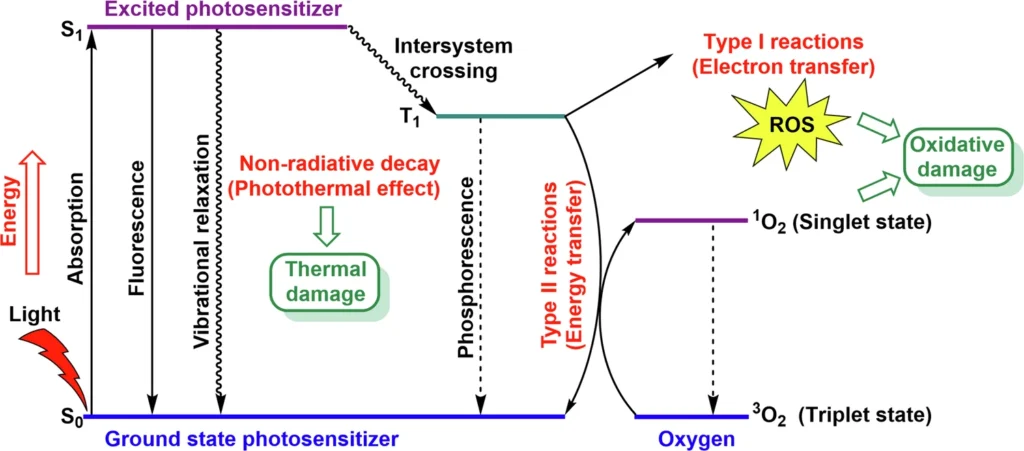
Cyanine dyes are highly effective in targeted therapy:
What Are Cyanine Dyes? Cyanine dyes are a type of synthetic colorant that belongs to the cyanine dye family. Many applications, including photography, printing, and biomedical research, commonly use them due to their deep blue or green color.
Cyanine dyes, a category of artificial dyes, find extensive application in several scientific and medical fields due to their vibrant hues and distinctive photophysical characteristics. These dyes feature a polymethine chain, a conjugated system of alternating double and single bonds, encircled by two nitrogen-containing heterocycles.
Cyanine dyes possess a unique characteristic that enables them to absorb light in the visible to near-infrared (NIR) areas. This property makes them highly valuable for applications that necessitate the ability to penetrate deep tissues with light, such as in vivo imaging and phototherapy.
Chemical structures and properties:
A polymethine chain, which can have varying lengths, composes cyanine dyes. The amount of conjugated double bonds in the chain determines the wavelength at which the dye absorbs light. As the length of the polymethine chain increases, the absorption peak shifts progressively towards the near-infrared (NIR) region. This characteristic is important in biomedical applications because near-infrared (NIR) light has greater tissue penetration capabilities than visible light. This allows for more accurate and effective targeting of cancers located beneath the skin.
Furthermore, cyanine dyes are very good at both absorbing light and giving off a lot of fluorescence. This makes them perfect for use as fluorescent markers in imaging. We can readily alter their chemical composition to enhance their resistance to light degradation, enhance their ability to dissolve in a liquid, and selectively bind to certain biological targets, thereby expanding their usefulness in various medical treatment and diagnostic scenarios.
Historical Evolution and Utilizations:
In the mid-19th century, scientists first synthesized cyanine dyes specifically for use in photographic emulsions. Over time, their uses broadened to encompass molecular biology, including tasks like labeling nucleic acids and proteins. The biomedical area has used cyanine dyes for several purposes, including the creation of fluorescent probes for imaging, biosensors, and diagnostic assays.
The identification of their phototherapy capacity was a significant breakthrough in the development of cyanine dyes. Researchers found that these dyes could serve as photosensitizers in photodynamic therapy (PDT). Near-infrared (NIR) light can trigger the dyes in this therapy, generating reactive oxygen species (ROS) that cause harmful effects in specific cells. This breakthrough has created new opportunities for utilizing cyanine dyes in cancer treatment, especially in therapies that require accurate targeting and low invasiveness.
Phototherapy’s mechanism of action:
Matter absorbs photons, which results in the transfer of energy:
In phototherapy, cyanine dyes work very well because they can absorb light in the near-infrared (NIR) range and send energy to other molecules very efficiently. When a cyanine dye absorbs light, it transitions to an excited state, which is a higher energy level compared to its ground state. When the dye is excited, it can transfer energy to molecules that are close by, which starts a sequence of photochemical processes.
Within the framework of photodynamic therapy (PDT), the cyanine dye in an excited state can transfer energy to molecular oxygen. This transfer leads to the production of singlet oxygen, which is a very reactive form of oxygen. Singlet oxygen, in addition to other reactive oxygen species (ROS), has the potential to harm different cellular constituents such as lipids, proteins, and nucleic acids, resulting in cell demise. This mode of operation is very beneficial in cancer treatment, as it enables the targeted elimination of malignant cells while preserving healthy tissues.
Production of Reactive Oxygen Species (ROS):
The production of reactive oxygen species (ROS) is a crucial mechanism via which cyanine dyes exhibit their therapeutic effects in photodynamic therapy (PDT). ROS refers to oxygen-containing compounds that are highly reactive, including superoxide anion, hydrogen peroxide, and hydroxyl radicals. These chemicals can cause oxidative stress in cells, which can lead to cell death if the level of oxidative damage exceeds the cell’s capacity for self-repair.
When cyanine dyes are turned on in cancer cells, they make too many reactive oxygen species (ROS), which can be stronger than the antioxidant defenses of the cells. This results in significant harm to mitochondria and other essential cellular components. Oxidative stress can induce apoptosis or necrosis, leading to the elimination of cancer cells. The capability to direct the cyanine dye to specific cellular compartments, such as the mitochondria, enhances the precision of this procedure, as the generation of reactive oxygen species (ROS) can significantly impact cell survival. Type of cyanine dyes.
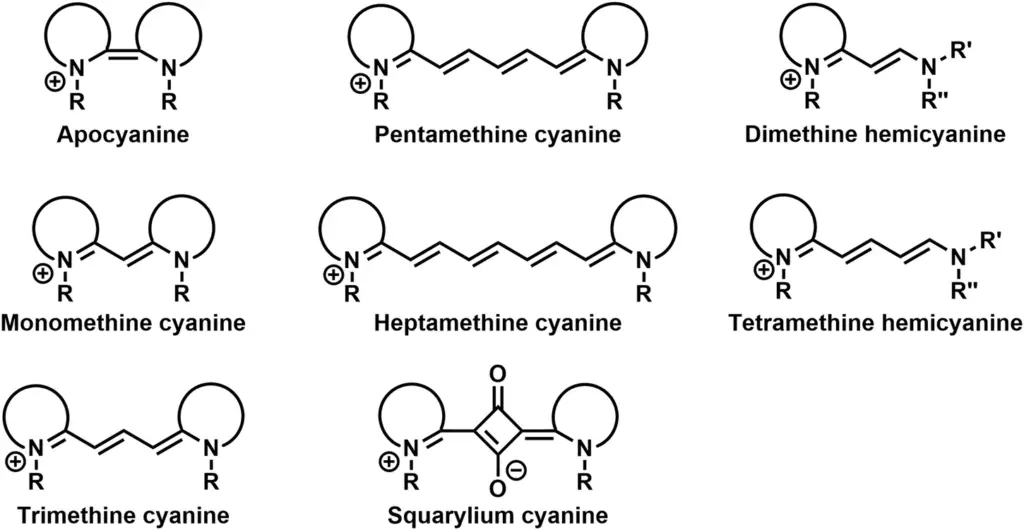
Cyanine dyes with improvement photostability.
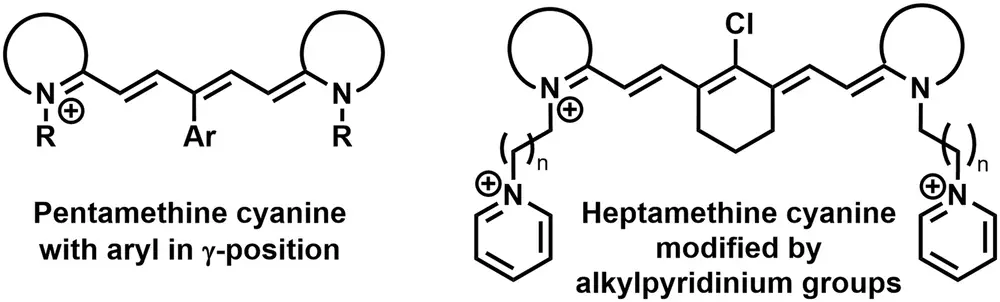
Cyanine dyes for targeting mitochondria:
Significance of Mitochondria Targeting:
The benefits of specifically targeting mitochondria in cancer cells are substantial.
Multiple factors make the method of targeting mitochondria highly advantageous in cancer therapy. Initially, cancer cells frequently depend extensively on mitochondrial activity to fulfill their energy requirements and sustain their rapid proliferation. Therapies that alter mitochondrial function can effectively deprive cancer cells of their energy supply, resulting in their demise.
Furthermore, mitochondria play a critical role in controlling apoptosis. Therapies that specifically target mitochondria can directly activate the apoptotic pathways, resulting in the regulated death of cancer cells. This strategy is especially beneficial in overcoming the resistance mechanisms that cancer cells build against traditional medicines, which frequently entail evading apoptosis.
Furthermore, by specifically targeting mitochondria, it becomes possible to selectively eliminate cancer cells while minimizing any adverse effects on nearby healthy organs. By leveraging the differences in mitochondrial membrane potential and function between malignant and non-malignant cells, we achieve this selectivity. It is possible to make cyanine dyes more selective by engineering them to concentrate in mitochondria. This makes them very useful for targeting cancer therapy.
Specificity of Cyanine Dyes for Mitochondria:
The selectivity of cyanine dyes for mitochondria is a crucial determinant of their efficacy as treatments. Engineers specifically engineer mitochondria-targeting cyanine dyes to accumulate in cancer cells’ mitochondria, leveraging the differences in membrane potential and lipid composition between diseased and non-cancerous cells. The process of selectively accumulating the phototherapeutic effects enables the targeted distribution of these effects specifically to the mitochondria. This targeted delivery maximizes the harm inflicted on the cancer cells.
The lipophilic cationic nature of cyanine dyes is a key characteristic that makes them particularly suitable for mitochondria. The mitochondrial membrane potential, which is more negative in cancer cells, allows these positively charged dyes to enter the mitochondria. Upon entering, the dyes can interact with the mitochondrial components, resulting in their retention and buildup. The ability to alter the chemical structure of cyanine dyes allows for the optimization of their affinity for mitochondrial membranes, thereby enhancing their selectivity.
We design and engineer cyanine dyes using a systematic process:
Enhancements for localizing mitochondria
Researchers have employed a variety of chemical changes to boost the localization and preservation of cyanine dyes within the mitochondria, thereby improving their ability to target this organelle. An effective method involves integrating lipophilic cations, such as triphenylphosphonium (TPP) groups, into the dye’s composition. These positively charged ions enhance the dye’s ability to pass through mitochondrial membranes and gather inside the organelle, propelled by the membrane’s negative electrical potential.
Another approach entails improving the dye’s charge distribution and hydrophobicity to increase its attraction to mitochondrial membranes. Scientists can produce cyanine dyes that specifically accumulate in the mitochondria by adjusting these characteristics, ensuring that the therapeutic impacts of PDT or PTT concentrate on the desired cells.
Additionally, researchers are exploring the use of targeted ligands, like peptides or small molecules, in conjunction with cyanine dyes. The ligands can increase the dye’s selectivity for specific types of cancer cells or subcellular compartments, thereby enhancing the precision of the therapy’s targeting.
Illustrations of Cyanine Dyes that Specifically Target Mitochondria:
Researchers have created and examined multiple cyanine dyes due to their specific capability to preferentially target mitochondria. An example is MitoTracker, a dye that is readily available for purchase and extensively used in scientific investigations to mark and see mitochondria within living cells. Due to their membrane potential, MitoTracker dyes specifically accumulate in the mitochondria, making them highly useful for investigating mitochondrial function and dynamics.
Another instance is Cy5.5, a near-infrared cyanine dye that has been utilized in diverse preclinical investigations for imaging and therapy. Changes to Cy5.5 can enhance its ability to target mitochondria, making it a compelling candidate for use in mitochondria-targeting photodynamic therapy (PDT) and photothermal therapy (PTT). Preclinical cancer models have shown substantial promise for these dyes, as they specifically accumulate in tumor mitochondria and trigger cell death when activated by light. Examples of Photodynamic cyanine dyes.
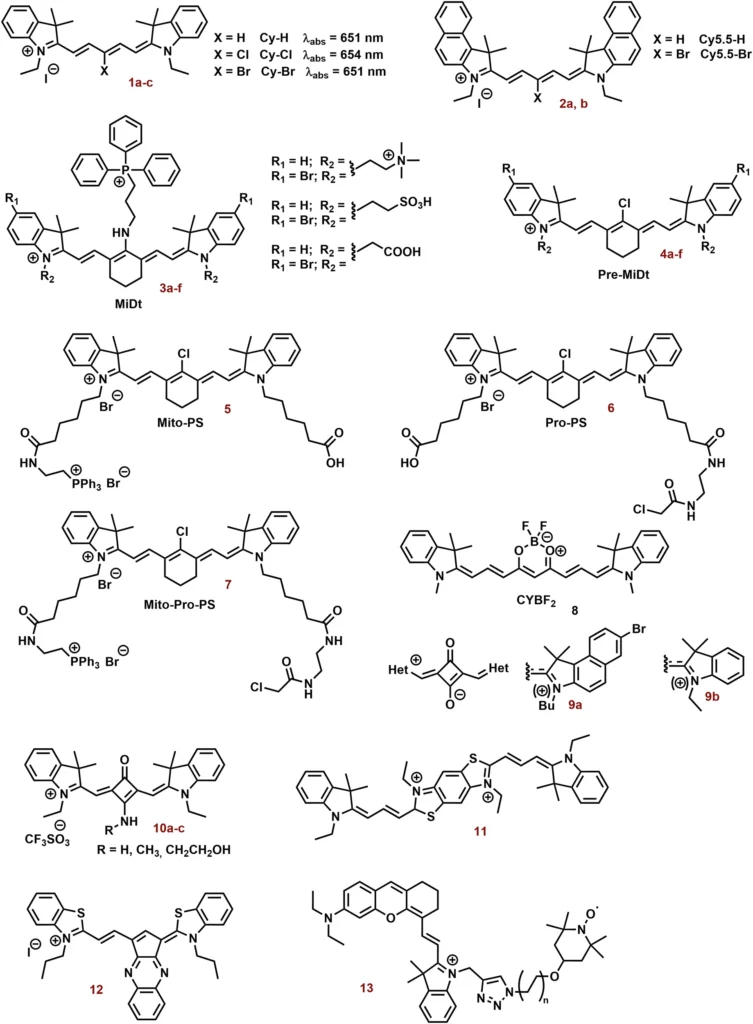
Utilizing Cyanine Dyes for Photodynamic Therapy (PDT):
The mechanism underlying photodynamic therapy (PDT) involves cyanine dyes.
The interaction of light and oxygen
Cyanine dyes are used in photodynamic therapy (PDT) to kill cancer cells by creating reactive oxygen species (ROS). The procedure commences by administering a cyanine dye, which specifically gathers in the mitochondria of cancerous cells. When a certain wavelength of light, usually in the near-infrared (NIR) range, hits the dye, it gets excited and transfers energy to nearby molecules of oxygen. This makes the oxygen change into singlet oxygen and other reactive oxygen species (ROS).
These reactive oxygen species (ROS) possess a high reactivity and can inflict significant harm on cellular constituents, such as lipids, proteins, and nucleic acids. The presence of reactive oxygen species (ROS) causes oxidative damage, resulting in the impairment of mitochondrial function, deterioration of membrane integrity, and eventually, the demise of cells. Because of its ability to trigger cell death via oxidative stress, PDT is highly effective in selectively targeting cancer cells, which frequently display increased susceptibility to ROS as a result of their modified redox equilibrium. Examples of multifunctional photodynamic dyes.
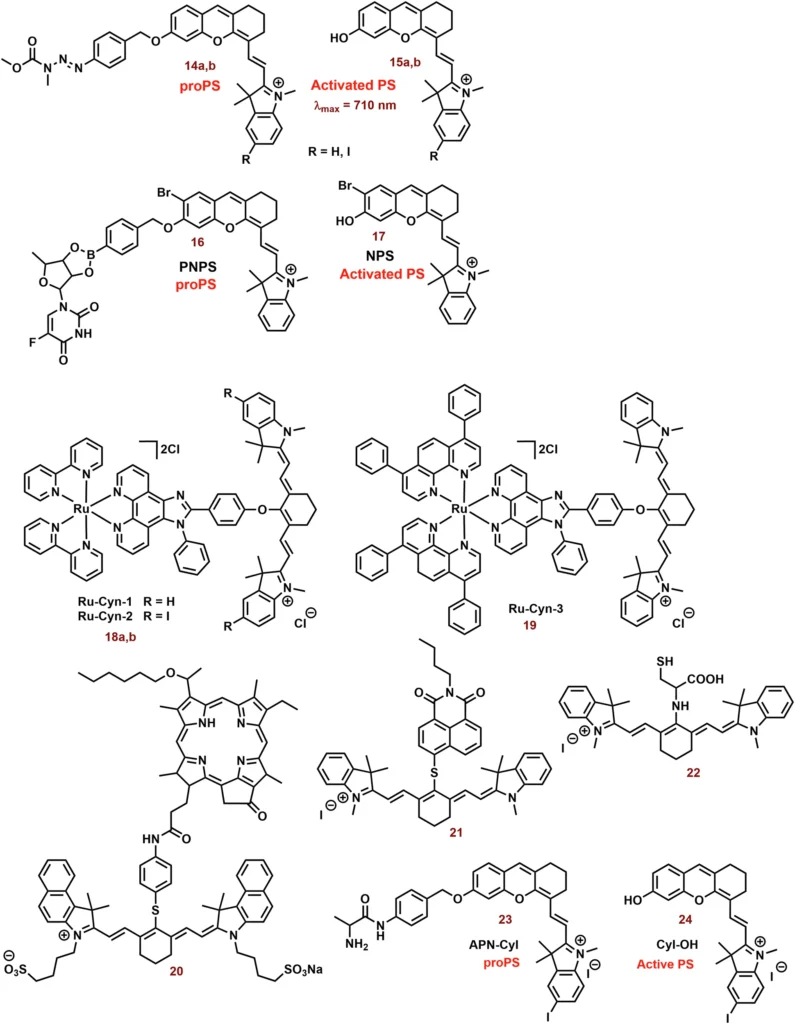
ROS induces pathways of cell death.
ROS production during PDT triggers multiple cellular death pathways, predominantly apoptosis and necrosis. Sometimes, during apoptosis, reactive oxygen species (ROS) damage the mitochondrial membrane. This lets pro-apoptotic substances like cytochrome c into the cytoplasm. The release of this substance initiates the activation of caspases, which are a group of enzymes that carry out the cell death process by cutting important cellular proteins.
ROS, in addition to apoptosis, can also trigger necrosis, which is a type of cell death characterized by the uncontrolled rupture of the cell membrane and the release of intracellular contents. Necrosis is commonly linked to inflammation and can enhance the immune response against the tumor. PDT’s capacity to trigger both apoptotic and necrotic cell death offers a versatile strategy for cancer treatment, enhancing the probability of total tumor elimination.
Applications in the Field of Cancer Treatment
We conduct examinations of specific instances and scientific experiments involving patients.
Photodynamic treatment with cyanine dyes has demonstrated potential in treating various forms of cancer, as evidenced by multiple case studies and clinical trials that have emphasized its effectiveness. For instance, photodynamic therapy (PDT) has effectively treated superficial and localized cancers, such as skin cancer, by allowing light to quickly penetrate and activate the photosensitizer. Clinical investigations have shown that patients with non-melanoma skin cancer have achieved a substantial reduction in tumor size and long-lasting absence of the disease after undergoing photodynamic therapy (PDT) using cyanine dyes.
Researchers have investigated photodynamic therapy (PDT) for treating interior tumors such as head and neck cancer, breast cancer, and lung cancer, in addition to its application in treating skin cancer. In such instances, fiber-optic devices frequently transport light to the tumor location, facilitating the activation of the photosensitizer in the body’s depths. Clinical investigations have substantiated the efficacy of PDT in diminishing tumor size, improving patient outcomes, and increasing the quality of life for individuals afflicted with recalcitrant malignancies.
Photodynamic Therapy (PDT) Using Cyanine Dyes: Benefits and Difficulties
An important benefit of PDT utilizing cyanine dyes is its capacity to specifically target and eradicate cancer cells while limiting harm to healthy tissues. Targeted dye accumulation in the tumor and accurate light administration to the therapy site synergistically achieve this selectivity. Moreover, PDT is a procedure that involves minimum invasion, hence decreasing the likelihood of problems and enabling a quicker recovery as compared to conventional surgical techniques.
Nevertheless, certain obstacles to PDT must be resolved to fully realize its potential. A major obstacle is the restricted ability of light to penetrate deeply, which can hinder the efficacy of PDT in addressing cancers that are big or located deep within the body. Scientists are investigating different approaches to address this constraint, including the advancement of more effective methods for delivering light and the use of NIR dyes that have improved the ability to penetrate tissues.
Another obstacle is the possibility of phototoxicity, which occurs when the activated dye can harm nearby tissues if not accurately directed. Current research concentrates on enhancing the selectivity of cyanine dyes for cancer cells and devising techniques to more accurately regulate the activation of the dye to reduce this risk. Anticancer effect of xanthenecyanine with DNA methylation ability.
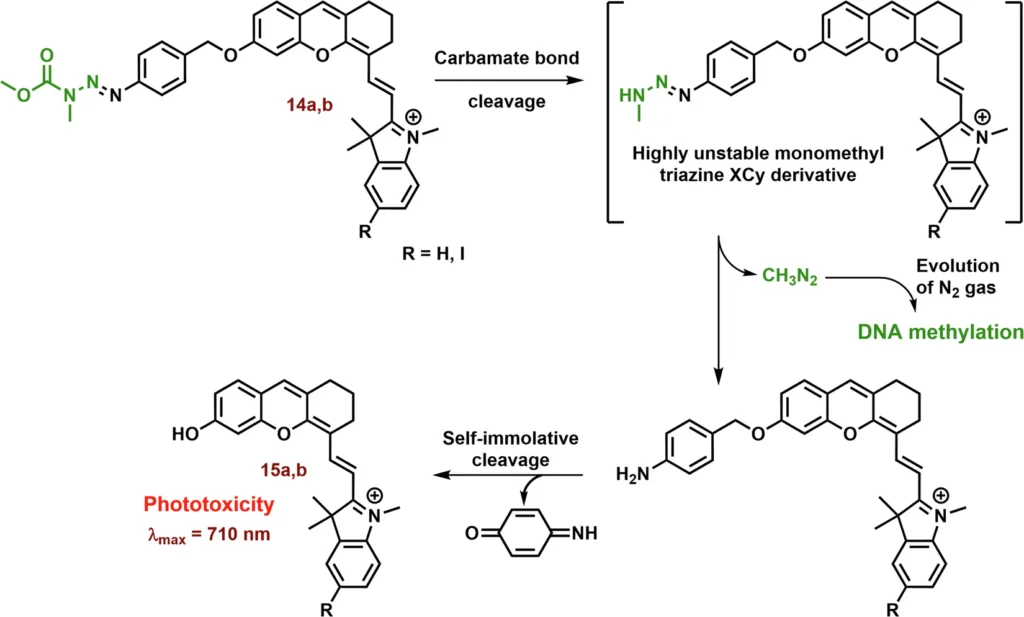
Utilizing Cyanine Dyes for Photothermal Therapy (PTT):
The process of photothermal therapy (PTT) incorporates cyanine dyes.
Thermal energy production and cellular annihilation
Photothermal therapy (PTT) with cyanine dyes is a promising method for treating cancer that exploits the dyes’ capacity to turn light into heat. When a cyanine dye encounters near-infrared (NIR) light, it absorbs the energy and converts it into heat through non-radiative relaxation mechanisms. The rise in temperature in a specific area can cause harm to cancer cells, such as altering the structure of proteins, disrupting cell membranes, and triggering programmed cell death or tissue death.
The effectiveness of photothermal therapy (PTT) depends on many things, including how well the dye absorbs light, how strong the light is, and how well it can produce enough heat to kill cells while protecting nearby healthy tissues. Cyanine dyes are very suitable for photothermal therapy (PTT) because they have a high absorption capacity in the near-infrared (NIR) range. This enables light to penetrate deeper into tissues, making it possible to treat cancers lying beneath the skin.
Integration with complementary treatment approaches
Combining PTT with other therapeutic modalities like chemotherapy, immunotherapy, or PDT can enhance the overall effectiveness of cancer treatment. During PTT, the heat produced can enhance the permeability of cancer cell membranes, facilitating the absorption of chemotherapy medicines. The synergistic impact mentioned can enhance the efficacy of tumor elimination and decrease the probability of cancer reoccurrence.
Combining PTT (photothermal therapy) with PDT (photodynamic therapy) creates a multi-modal method that specifically targets cancer cells. This strategy utilizes both thermal and oxidative stress, hence enhancing the likelihood of destroying the tumor. Combining these therapies reduces the required dosages for each treatment, thereby minimizing adverse effects and improving patient outcomes. Conjugate of cyanine dye with fluorouridine cytostatic.
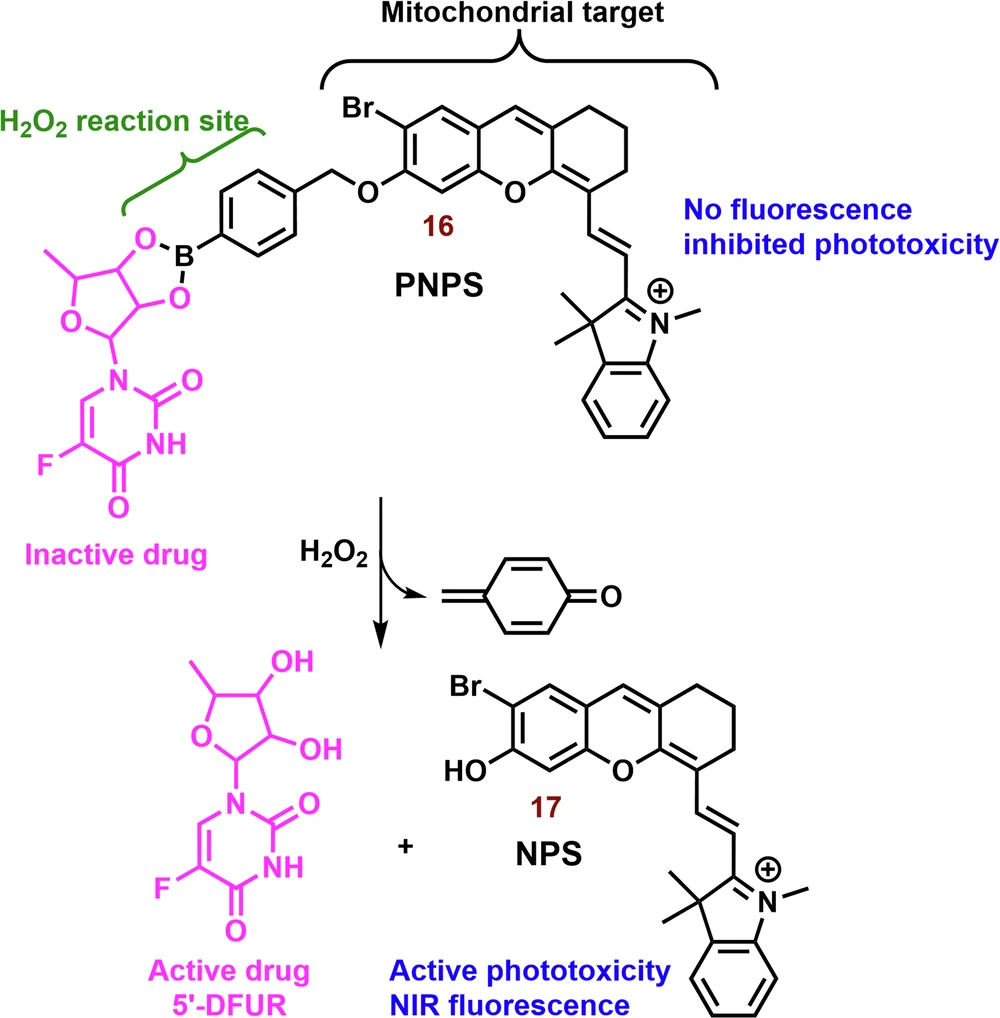
Interaction of cyanine probe with cysteine.
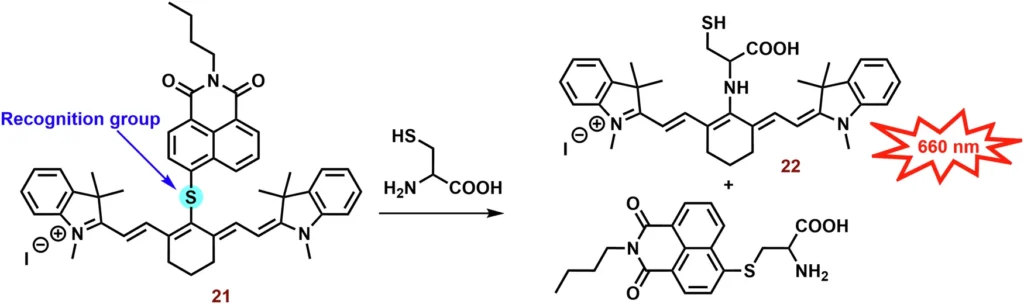
Utilizations in Cancer Therapy:
We conduct examinations of specific instances and scientific experiments involving patients.
Preclinical and clinical studies have investigated the use of cyanine dyes in photothermal treatment, revealing its promise for treating diverse types of malignancies. Animal models have demonstrated that PTT is highly successful in decreasing tumor size and impeding tumor progression, especially when used in conjunction with other treatments. Studies on prostate cancer and glioblastoma have demonstrated that cyanine dye-based photothermal therapy (PTT) can effectively destroy tumors while causing minimal adverse effects.
Ongoing clinical trials are investigating the use of PTT in cancer patients, and initial findings indicate promising potential. PTT has been tested in breast cancer patients to selectively target and eliminate remaining tumor cells after surgery. These trials have showcased the efficacy of PTT in enhancing patient outcomes and mitigating the likelihood of cancer recurrence, especially in situations where traditional treatments have limited efficacy.
The Advantages and Difficulties of PTT Using Cyanine Dyes:
Using cyanine dyes in PTT has many benefits, including being very specific, not causing too much damage, and being able to target tumors that don’t respond to other treatments. By manipulating the light settings, one may exert exact control over PTT, enabling the targeted eradication of cancer cells while safeguarding healthy tissue. Furthermore, the use of NIR light improves the ability to penetrate tissues, making photothermal therapy (PTT) suitable for treating malignancies located at greater depths.
Nevertheless, there are still obstacles to overcome to optimize PTT for therapeutic applications. One obstacle that arises is the need for meticulous regulation of heat production in order to prevent harm to adjacent healthy tissues. For this, accurate light dosage adjustment and continuous temperature monitoring in the treatment zone are necessary. Another obstacle is the possibility of inadequate tumor coverage, especially in tumors that are big or have irregular shapes. Scientists are tackling these difficulties by creating sophisticated imaging methods and monitoring systems that can direct the use of PTT and guarantee thorough tumor therapy.
The synergistic effects of combining photodynamic therapy (PDT) and photothermal therapy (PTT):
Advantages of Combined Therapy
Improved efficacy in cancer treatment
The combination of photodynamic therapy (PDT) and photothermal therapy (PTT) provides numerous advantages that can improve the overall effectiveness of cancer treatment. An important benefit is the capacity to specifically target cancer cells using various methods, hence enhancing the chances of eliminating the tumor. PDT causes cell death by inducing oxidative stress through the production of reactive oxygen species (ROS), while PTT eliminates cancer cells by generating localized heat. The integration of these two techniques can result in a more resilient and all-encompassing approach to cancer treatment.
Moreover, the collaborative effects of merging photodynamic therapy (PDT) and photothermal therapy (PTT) can amplify the responsiveness of cancer cells to treatment. For instance, the reactive oxygen species (ROS) produced during photodynamic therapy (PDT) might enhance the sensitivity of cancer cells to heat, hence increasing their vulnerability to the effects of photothermal therapy (PTT). This heightened sensitivity can lead to decreased dosages of each treatment, minimizing the likelihood of adverse reactions and enhancing patient results. Functionality of Hcyanine dyes in dependence of pH.
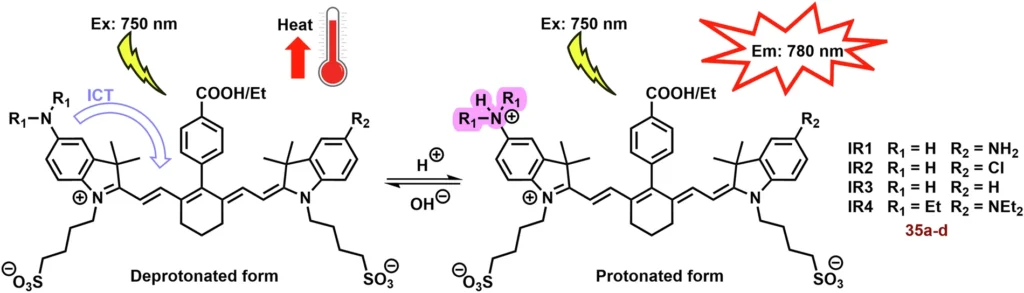
Decrease in Adverse Effects:
Another notable benefit of integrating PDT and PTT is the ability to reduce adverse effects. Combining reduced dosages of each medication can potentially reduce the overall treatment load on the patient. This method additionally enables more accurate localization of cancer cells, hence minimizing the effect on healthy tissues. Customizing the treatment based on the tumor’s attributes, such as its location, size, and kind, can also lead to a decrease in adverse effects and enhance the overall quality of life for patients receiving therapy.
Analysis of Present Studies and Prospective Avenues for Investigation:
The design of cyanine dyes has advanced significantly.
The primary focus of ongoing research in the field of cyanine dye-based phototherapy is to create novel dyes with superior photophysical properties and more effective targeting abilities. The goal of dye design innovations is to improve the stability, solubility, and photostability of cyanine dyes, thereby increasing their efficacy in therapeutic applications. In addition, scientists are investigating the application of nanotechnology to improve the delivery of cyanine dyes to tumors, thereby increasing their concentration and duration within cancer cells.
The development of flexible dyes, which serve a variety of purposes in both photodynamic therapy (PDT) and photothermal therapy (PTT), is another area of study. This enables a more comprehensive and adaptable strategy for the treatment of cancer. Specifically engineered to respond to various wavelengths of light, these dyes enable the targeted activation of either photodynamic therapy (PDT) or photothermal therapy (PTT), based on the patient’s requirements. The capacity to alternate between therapies or employ them in conjunction is a potent instrument for personalized therapy. Phototoxicity of HC-1 (compound 17) on the dependence of light irradiation wavelength.
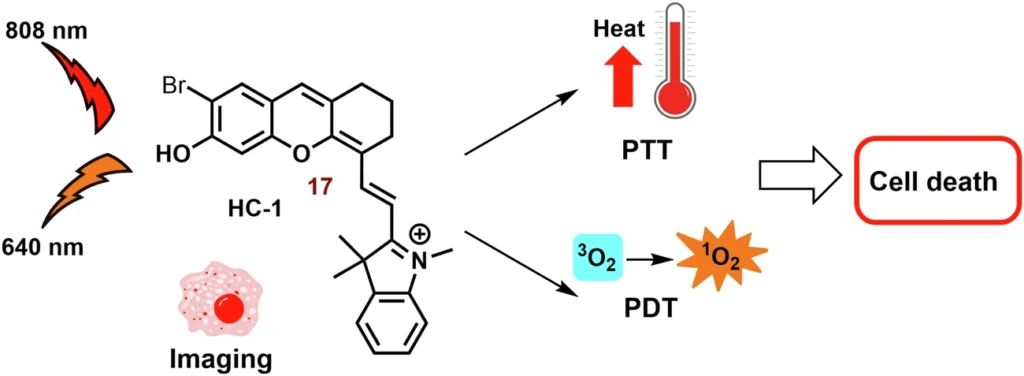
Potential for Individualized Medicine:
As our knowledge of cancer biology advances, there is a growing possibility of utilizing cyanine dye-based therapies for personalized medicine. Personalized medicine is the practice of customizing treatment based on specific characteristics of a patient’s tumor, including genetic alterations, metabolic profile, and tumor microenvironment. We can easily alter the chemical composition of cyanine dyes to specifically target specific tumor attributes, offering a distinct possibility for individualized therapy approaches.
Researchers are currently exploring the potential of targeting specific receptors or proteins in cancer cells using targeted ligands or antibodies linked to cyanine dyes. This method enables the accurate administration of phototherapeutic effects on the tumor, minimizing unintended consequences and enhancing the treatment’s effectiveness. Furthermore, the ability to monitor treatment response in real-time using imaging techniques could provide crucial insights into the therapy’s effectiveness, allowing for the implementation of necessary modifications.
The future of cancer care could include cyanine dye-based medicines in personalized treatment programs, offering a more customized and efficient approach. As scientific research goes on, new dyes and delivery methods will be developed that will make these therapies even better at helping patients and giving them a more accurate and minimally invasive way to treat their cancer.
In conclusion:
Cyanine dyes have become a potent weapon in the realm of mitochondria-targeting photodynamic and photothermal therapy, presenting a hopeful avenue for cancer treatment. Because they have the unique ability to gather only in mitochondria and they behave differently under light, they are strong candidates for killing cancer cells precisely. The development of novel cyanine dyes and their use in both photodynamic therapy (PDT) and photothermal therapy (PTT) have created new opportunities for cancer treatment, offering a more accurate and less intrusive alternative for targeting tumors.
Using both photodynamic therapy (PDT) and photothermal therapy (PTT), made easier by cyanine dyes, together is a method that benefits everyone because it makes treatment more effective while lowering the risk of side effects. Research and advancements in dye design and delivery systems continuously push the boundaries of phototherapy, leading to the creation of more personalized and effective cancer treatments.
Looking ahead, the prospect of incorporating cyanine dye-based medicines into tailored treatment plans is very thrilling. By customizing the treatment based on the unique attributes of a patient’s tumor, we can provide a more focused and less intrusive method of cancer care, enhancing patient results and offering renewed optimism in the battle against this destructive illness.
Frequently Asked Questions:
1). What characteristics make cyanine dyes well-suited for mitochondria-targeting therapy?
Because they can absorb light in the near-infrared region, modify their structure to increase specificity, and effectively produce reactive oxygen species and heat to destroy targeted cells, cyanine dyes are well-suited for mitochondria-targeting therapy.
2). What are the distinguishing characteristics between photodynamic therapy and photothermal therapy?
Photodynamic therapy uses light to activate photosensitizers that make reactive oxygen species, which kill cells. Photothermal therapy, on the other hand, uses light-absorbing compounds to make heat, which kills cancer cells in a specific area.
3). Are there any side effects associated with cyanine dye-based therapies?
Although cyanine dye-based therapies are generally well-tolerated, there is a possibility of experiencing localized tissue damage due to heat in photothermal therapy (PTT) and oxidative stress in photodynamic therapy (PDT). However, these effects typically only affect the specific region under treatment.
4). What are the potential outcomes of ongoing cyanine dye research in the field of cancer treatment?
The future of cyanine dye research in cancer treatment includes making dyes that are more precise and effective, adding medicines to make them work better, and looking into how to make treatment plans that are specific to each tumor.
5). Is it possible to customize cyanine dye-based treatments for particular patients?
Indeed, it is possible to customize cyanine dye-based therapies by adapting the treatment to the precise molecular attributes of a patient’s tumor. This approach has the potential to enhance effectiveness and minimize adverse reactions.
For more chemistry blogs, visit chemistry Master