Table of Contents
Phosphorescence is a fascinating occurrence that has captivated scientists for many years. Materials’ luminescent properties, which enable them to emit light upon illumination, have led to a diverse array of practical applications such as glow-in-the-dark toys and advanced bioimaging techniques. Historically, we have mostly artificially created phosphorescent materials, often containing scarce or hazardous components. Recent research has revealed that lignin, a natural polymer, can display photoactivated room-temperature phosphorescence (RTP). This discovery of Phosphorescence has the potential to completely transform the field by providing a sustainable and environmentally acceptable alternative to traditional phosphorescent materials.
Comprehending the Basic Principles of Phosphorescence:
Phosphorescence is a form of photoluminescence in which a substance absorbs light particles (photons) and subsequently emits them over a prolonged duration. Phosphorescence, in contrast to fluorescence, is characterized by a delayed emission that can persist from milliseconds to many hours after absorption.
Phosphorescence is based on quantum mechanics principles. When a substance absorbs light, its electrons become energized and move to a higher energy state. Quantum mechanics considers phosphorescent materials to confine electrons in a metastable triplet state, a “forbidden” state. This triplet form returns to the ground state at a sluggish pace, resulting in the delayed release of light.
Phosphorescence exhibits notable distinctions from fluorescence. In fluorescence, the excited electrons quickly return to their initial energy level, prompting the emission of light. Typically, this process concludes in nanoseconds. An energy barrier confines the electrons in the triplet state during phosphorescence, impeding their rapid return to the ground state. This energy barrier allows phosphorescence to persist for an extended period, even after the removal of the light source.
Phosphorescence necessitates precise environmental and chemical conditions. The material must possess the appropriate electronic configuration to facilitate the creation of a triplet state. Furthermore, the surrounding environment must be conducive to maintaining the phosphorescence without extinguishing it. For example, oxygen, which is a quencher in a triplet state, can inhibit phosphorescence by interacting with the excited electrons, enabling them to transition back to the ground state without generating light. Thus, to sustain their luminescence, phosphorescent materials frequently require either an atmosphere devoid of oxygen or the application of protective coatings.
In the past, inorganic compounds, like copper-doped zinc sulfide, or organic molecules, like phosphorescent pigments, typically composed phosphorescent materials. Several domains, from emergency exit signs to luminescent paints, have utilized these materials. In their quest to find novel phosphorescent materials that can function at ambient temperature while also being environmentally friendly, researchers have been investigating natural polymers such as lignin. Schematic illustrating the preparation and properties of PLA-Lig.
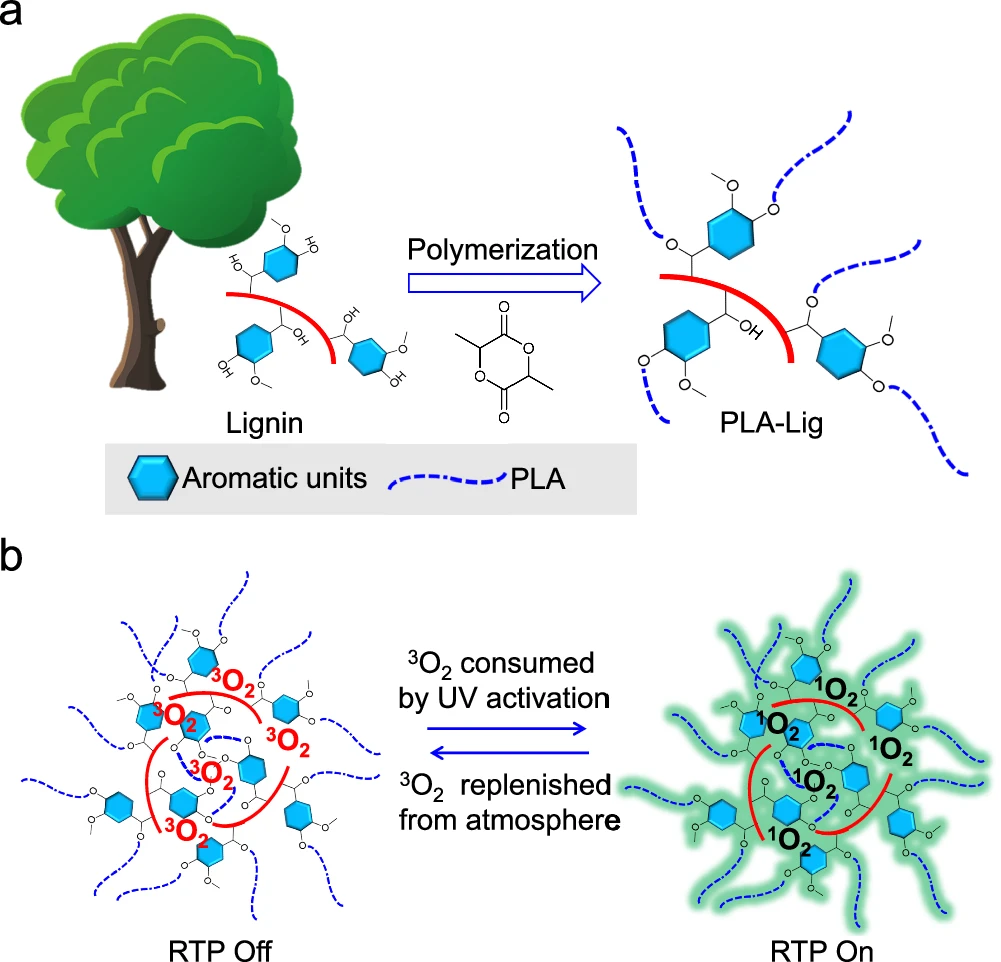
Lignin’s Function in Nature and Industry:
Lignin is a highly prevalent biopolymer on Earth, ranking second only to cellulose in terms of abundance. It is an intricate, non-uniform polymer mostly consisting of phenolic chemicals, which are aromatic alcohols. Lignin is predominantly present in the cellular walls of plants, serving a vital function in offering structural reinforcement, stiffness, and protection against microbial assault.
Lignin functions as an adhesive for cellulose fibers in plants, providing structural support to the cell walls and enhancing their resistance to different environmental pressures. It is especially prevalent in woody plants, where it enhances the robustness and longevity of the wood. Lignin plays a vital role in facilitating water transportation within plants by providing essential structural support for the vascular tissues responsible for carrying water and nutrients from the roots to the leaves.
In addition to its biological function, lignin plays a crucial role in the global carbon cycle. Plants absorb carbon dioxide from the environment as they grow, transforming it into organic materials, such as lignin. When plants die and decompose, they release the carbon in lignin into the atmosphere or assimilate it into the soil, thereby contributing to the carbon cycle. The intricate composition of lignin renders it very resistant to degradation, resulting in its long-lasting presence in the environment and its consequential impact on carbon storage in soils.
Lignin is a byproduct of the industrial environment’s paper and pulp industry. The process of creating paper extracts it from cellulose. Historically, pulp mills frequently incinerate lignin as a low-grade fuel, viewing it as a byproduct with little value. Nevertheless, there is a shift in perception as scientists and businesses acknowledge the potential of lignin as a rich source of chemicals and materials.
Lignin has the potential to generate a diverse array of products, including carbon fibers, biofuels, adhesives, and bioplastics. Owing to its aromatic structure, lignin can serve as a source of phenolic compounds used in the production of resins, plastics, and other chemicals. Moreover, lignin’s high prevalence and replenishment ability make it an appealing primary substance for the creation of environmentally friendly, biologically derived goods.
Although lignin valorization holds promise, it encounters many obstacles. An important challenge is the variability of lignin, which exhibits variations based on its source (such as wood, grass, or agricultural leftovers) and the extraction method employed. The diversity in lignin-derived products might impact their quality and attributes, which presents difficulties in establishing standardized techniques for large-scale production. Nevertheless, progress in the field of lignin chemistry and processing technologies is aiding in the resolution of these difficulties, hence creating fresh prospects for the utilization of lignin invaluable applications. Optical properties of PLA-Lig.
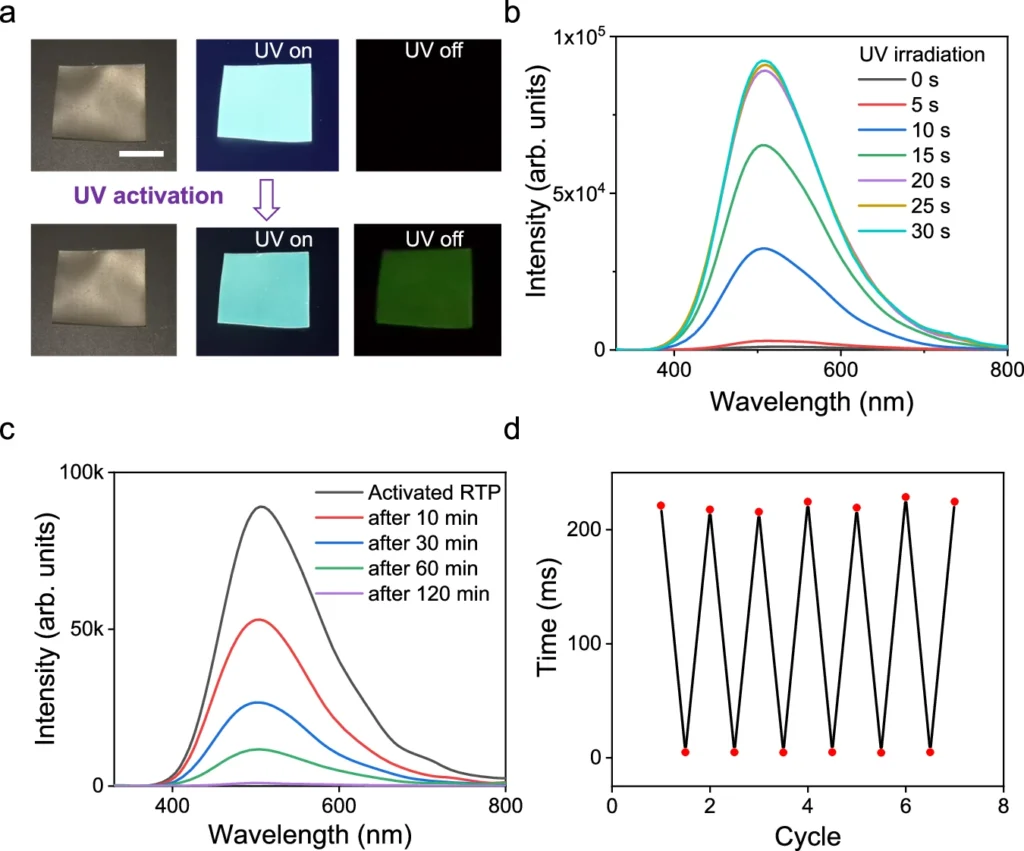
Exploring the phenomenon of photoactivated room temperature phosphorescence (RTP):
Room temperature phosphorescence (RTP) is the phenomenon when a material can emit phosphorescent light at normal temperatures. This is particularly difficult since, at normal temperature, thermal energy frequently causes the excited electrons to rapidly revert to their original state, extinguishing the phosphorescent glow. Because of this, Room temperature phosphorescence (RTP) materials need to have special properties that allow them to keep the charged electrons in the triplet state for long periods, even when the temperature is normal at room temperature.
Photoactivation is a crucial mechanism in Room temperature phosphorescence (RTP). It entails using light, usually ultraviolet or visible light, to stimulate the electrons in a substance to elevated energy states. Within Room temperature phosphorescence (RTP) materials, excited electrons become trapped in the triplet state. From this state, they gradually transition back to the ground state, releasing light as they do so. Exposure to light triggers the phosphorescence, as the term “photoactivated” indicates.
The significance of Room temperature phosphorescence (RTP) lies in its ability to be used across a wide range of domains. Conventional phosphorescent materials typically require low temperatures or certain conditions to display phosphorescence, which limits their practical application. On the contrary, Room temperature phosphorescence (RTP) materials can operate in regular settings, which makes them appropriate for a broad spectrum of uses, including bioimaging, sensors, security inks, and emergency illumination.
The identification of RTP in lignin is particularly thrilling, as it presents the opportunity to use a naturally occurring, plentiful, and sustainable substance in applications that traditionally depend on artificial phosphors. Furthermore, lignin’s biodegradability and low environmental footprint make it a compelling substitute for conventional phosphorescent substances, which may possess toxicity or lack biodegradability.
Phosphorescence mechanisms in lignin:
Lignin’s distinctive chemical composition, especially at room temperature, contributes to its phosphorescent properties. Lignin consists of an intricate arrangement of aromatic rings joined together by different connections, resulting in a densely interconnected, three-dimensional polymer. This structure’s presence is critical for the manifestation of its phosphorescent characteristics.
Exposure to light causes lignin to absorb energy, resulting in the excitation of its electrons and their transition to higher energy levels. Typically, in the majority of materials, these energized electrons rapidly revert to their original state, releasing light in the form of fluorescence. Nevertheless, within lignin, the electrons can become ensnared in a metastable triplet state, where they persist for a prolonged duration before reverting to the ground state. The appearance of phosphorescence is a result of this delayed return.
The aromatic structure of lignin enables the requisite electronic transitions, thereby facilitating the development of the triplet state. The intricate nature of lignin’s structure, characterized by its diverse array of bonds and aromatic units, results in a broad spectrum of energy levels. As a result, the stimulated electrons become trapped in the triplet state. To optimize lignin’s phosphorescent capabilities, it is important to prevent the presence of oxygen or other quenching agents. You can achieve this by maintaining an oxygen-free atmosphere or by applying protective coatings.
Photoactivation is essential for lignin phosphorescence. When light interacts with lignin, the aromatic rings in lignin absorb the energy, causing the electrons to get excited and triggering the phosphorescence process. Various elements, such as the wavelength of the activating light, the unique structure of the lignin, and the surrounding environmental conditions, influence the strength and length of the phosphorescence.
The inherent diversity of lignin also affects its phosphorescent properties. Due to its non-uniform nature, different samples of lignin can display diverse phosphorescent characteristics. The variety of lignin presents both problems and chances because it lets the phosphorescent properties be changed by choosing or changing certain types of lignin.
Investigation of Lignin RTP Via Experimental Studies:
Recent experimental research has focused on understanding and improving lignin’s RTP (residence time distribution) features. Scientists have employed a variety of methods to quantify and examine lignin’s phosphorescent properties, to reveal its fundamental mechanisms and uses.
Researchers use time-resolved photoluminescence spectroscopy as a key technique to investigate lignin RTP. This method enables researchers to monitor the gradual release of light from lignin following its exposure to a light source. Through the examination of the decay curve of the emitted light, scientists can ascertain the duration of the phosphorescence and acquire knowledge about the mechanisms that regulate it.
Researchers have employed UV-Vis absorption spectroscopy and infrared spectroscopy to investigate the electronic structure of lignin and its correlation with its phosphorescent characteristics. These strategies help researchers understand how the intricate structure of lignin affects its ability to capture and emit light energy.
The research has revealed that lignin can demonstrate room-temperature phosphorescence (RTP) under specific circumstances. For instance, the duration of phosphorescence in lignin varies from a few milliseconds to several seconds. Factors such as the lignin’s origin, the extraction process, and the climatic conditions during the experiment influence this variability. Scientists have found that changing some chemicals in lignin, like adding metal ions, can make its RTP properties better by keeping the triplet state stable and making the phosphorescence last longer.
Case studies on lignin RTP research have proven its promise in a wide range of applications. Researchers have suggested lignin-based RTP materials as potential options for bioimaging applications. These materials could function as biodegradable and non-toxic substitutes for conventional phosphorescent probes. Researchers have further explored the use of lignin RTP in environmental monitoring to identify changes in environmental parameters like pH levels or the presence of specific compounds.
Utilizations of Lignin-Based Renewable Thermoplastic Polymers:
Researchers are currently investigating the numerous possibilities for the utilization of RTP in lignin.
Biomedical Applications:
Bioimaging has the potential to use lignin-derived RTP materials, as their ability to emit light for an extended period can be beneficial for non-invasive imaging techniques. Lignin, unlike conventional phosphorescent probes, possesses the desirable qualities of being biodegradable and non-toxic, which makes it a highly appealing choice for medical applications. For instance, imaging methodologies can employ lignin RTP to trace cell mobility or observe biological activities over time.
Monitor the environment:
Sensors specifically engineered to detect alterations in the environment could utilize the thermoplastic properties of lignin. We can employ lignin-based RTP sensors to monitor soil conditions, measure pollution levels, or identify specific compounds in the environment. Lignin’s sustainability and biodegradability make it ideal for environmental applications, prioritizing environmental impact minimization.
Energy Harvesting and Storage:
Energy harvesting and storage systems may also utilize lignin-based RTP materials. For instance, their ability to absorb and emit light for a prolonged duration could enhance the effectiveness of solar cells or light-harvesting devices. Furthermore, the distinctive features of lignin-based RTP materials could potentially enhance the performance of energy storage devices like batteries or capacitors.
Forensics:
Lignin-based RTP materials have the potential to improve evidence detection and processing in forensic science investigations conducted at crime scenes. The production of phosphorescent markers could potentially utilize these materials. UV light can activate these markers, which are not visible under normal lighting conditions, to reveal hidden evidence. This could prove particularly beneficial in scenarios where traditional forensic techniques face limitations.
Uses in Agriculture:
Agriculture has the potential to utilize lignin RTP for monitoring plant health and soil conditions. For instance, farmers could use lignin-made sensors to monitor nutrient levels, soil pH, or moisture content, providing valuable data to improve crop growth. Incorporating biodegradable lignin RTP materials in agriculture would effectively mitigate the environmental consequences of farming techniques.
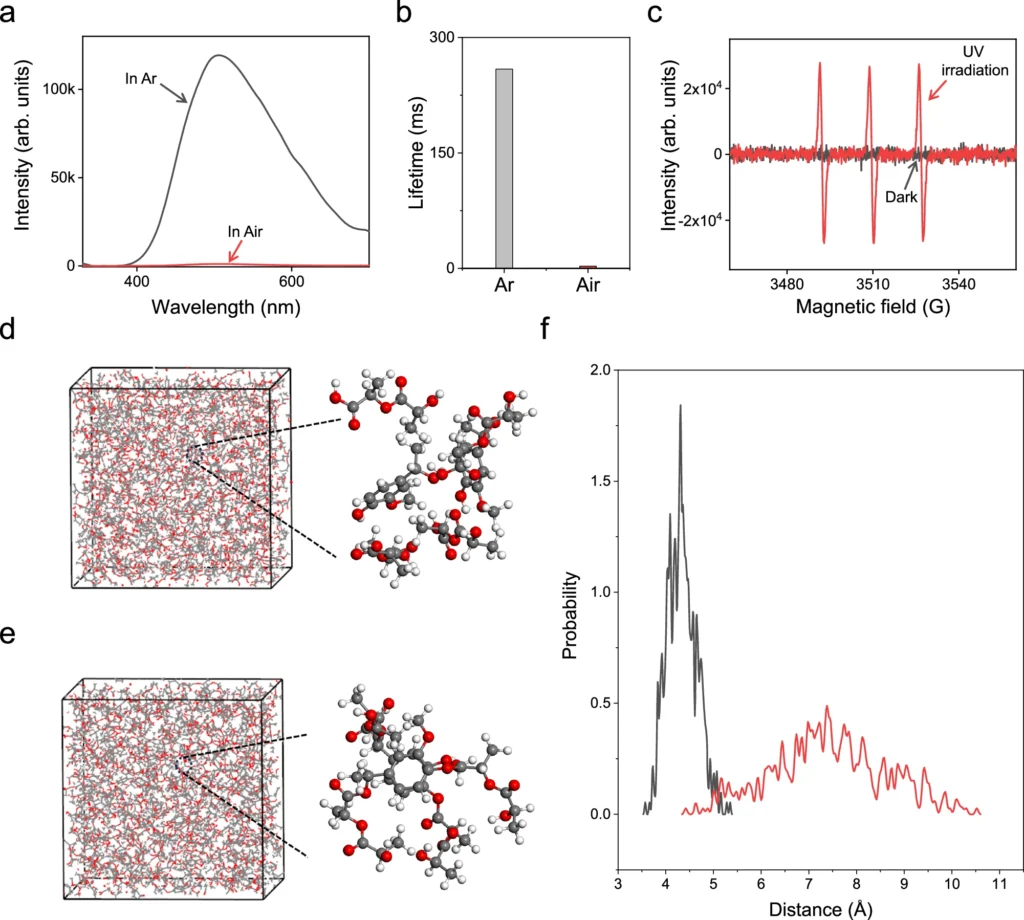
The lignin RTP’s sustainability and environmental impact are significant:
The sustainability of lignin makes it a highly desirable material for RTP applications. Lignin, a natural polymer, is readily available and renewable, especially as a byproduct of the paper and pulp industry. Applying lignin to high-value applications like RTP not only adds value to what would otherwise be considered waste but also promotes the progress of sustainable materials.
The biodegradability of lignin-based RTP materials significantly influences their environmental impact. Contrary to manmade phosphorescent compounds, which can endure in the environment for extended durations and potentially cause pollution, lignin is biodegradable and decomposes spontaneously over time. Consequently, lignin-based RTP materials have a lower tendency to collect in the environment, thereby diminishing their long-term ecological consequences.
Life cycle analysis (LCA) is a helpful method for evaluating the ecological consequences of lignin RTP in comparison to synthetic alternatives. Life Cycle Assessment (LCA) takes into account the complete life cycle of a substance, encompassing activities such as the extraction and processing of raw materials, as well as its use and disposal. The life cycle assessment (LCA) of lignin RTP probably shows that it has less of an effect on the environment than synthetic phosphors, especially when it comes to carbon emissions, resource use, and waste production.
Leveraging lignin RTP materials could potentially contribute to the advancement of a circular economy. In a circular economy, the goal is to maximize material utilization while minimizing waste and resource consumption. Industries can achieve a more sustainable production model by discovering novel applications for lignin, especially in valuable areas like RTP, which would result in waste reduction.
However, the widespread use of lignin RTP also poses potential ecological hazards that require attention. For instance, while natural processes can break down lignin, it’s crucial to investigate the byproducts of lignin-based RTP materials to prevent any detrimental effects on the environment or human health. Furthermore, it is critical to improve the production processes for lignin RTP materials to limit energy consumption and chemical usage, thereby further minimizing their environmental impact.
Benefits of Lignin in Phosphorescent Applications:
Lignin possesses numerous benefits compared to artificial phosphorescent substances, rendering it a highly appealing choice for a diverse array of uses.
Economic efficiency:
A major benefit of lignin is its cost-effectiveness. Lignin is a widely available and inexpensive byproduct of the paper and pulp industry. As a result, it becomes a cost-efficient substitute for synthetic phosphors, which frequently necessitate costly raw materials and intricate manufacturing procedures.
Frequency of occurrence in nature:
Earth abundantly contains lignin, a biopolymer that is readily available for use in a wide range of applications. The ample availability of lignin-based products guarantees a reliable supply chain, mitigating the potential for shortages that may arise with alternative materials.
Sustainability:
Lignin, being a natural and biodegradable substance, is ecologically sustainable. It has a smaller carbon footprint compared to manufactured phosphors and does not contribute to persistent environmental contamination. Lignin RTP materials are appealing to enterprises seeking to minimize their ecological footprint.
Processing flexibility:
The intricate composition of lignin permits a diverse array of chemical alterations and processing methods, facilitating the tailoring of its characteristics to suit certain uses. For instance, one can enhance the phosphorescent characteristics of lignin by adding metal ions or mixing it with other polymers. Flexible processing of lignin allows for the creation of customized products that meet the specific needs of various industries.
Biocompatibility and safety:
Lignin possesses non-toxic and biocompatible properties, rendering it appropriate for utilization in biomedical applications. Medical devices, medication delivery systems, and other healthcare applications deem lignin-based RTP materials safe for use, unlike certain artificial phosphors that may pose risks to human well-being.
Obstacles in harnessing lignin for RTP:
Although lignin offers numerous benefits, its use for RTP also poses a variety of obstacles that must be resolved.
Managing the emission of phosphorescent light:
Regulating lignin’s phosphorescent properties is a primary obstacle to using it for RTP. The intricate and diverse composition of lignin can result in variations in its phosphorescent characteristics, making it difficult to achieve uniform results. Scientists are now studying the variables that influence lignin’s phosphorescence and devising techniques to manipulate and enhance it for specific uses.
Lignin structure variability:
The structure of lignin is variable and depends on both its origin and the extraction technique used. The heterogeneity of lignin could affect its phosphorescent characteristics, making it difficult to standardize lignin RTP materials for commercial use. To address this obstacle, it is critical to devise techniques for characterizing and standardizing lignin.
Room temperature stability:
Although lignin can display room-temperature phosphorescence (RTP), maintaining a consistent phosphorescent state at normal room temperatures can be difficult. Environmental conditions, such as temperature and humidity, can influence the phosphorescent characteristics of lignin, potentially restricting its practical application. Scientists are investigating different approaches, such as chemical alterations and protective layers, to improve the durability of lignin RTP materials.
Large-Scale Production:
Transitioning the manufacturing of lignin RTP materials from the laboratory to an industrial scale poses numerous obstacles. These tasks encompass enhancing the extraction and processing techniques to guarantee uniform quality, devising streamlined manufacturing procedures, and tackling potential regulatory obstacles. It is crucial to overcome these hurdles to successfully introduce lignin RTP products to the market.
Longevity and durability:
The endurance and durability of lignin RTP materials are crucial factors, especially for applications that demand long-term stability. For the success of lignin RTP materials in commercial applications, they must retain their phosphorescent characteristics for long periods of environmental conditions. Applications.
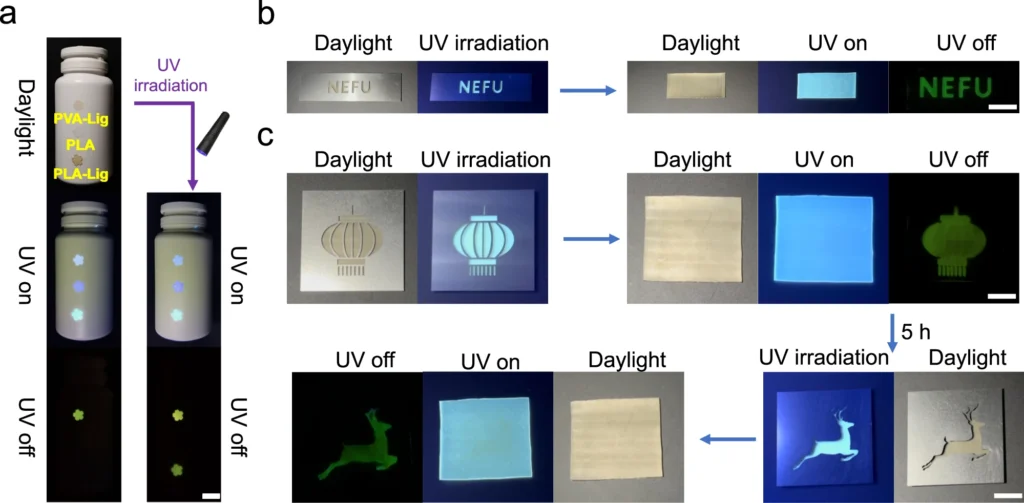
Modifications to Enhance Lignin’s Thermal Performance:
To overcome the accompanying obstacles, researchers are investigating various chemical and structural modifications to improve the phosphorescent capabilities of lignin RTP.
Metal Ion Doping:
Doping lignin with metal ions like copper or zinc enhances its phosphorescent properties. We achieve this by stabilizing the triplet state and extending the lifespan of phosphorescence. The metal ions interact with the lignin structure, generating additional energy levels. These energy levels facilitate the prolonged retention of excited electrons in the triplet state. This technique has demonstrated potential for improving lignin’s RTP (resistance to thermal degradation) properties, thereby increasing its suitability for real-world applications.
Blending with Other Polymers:
Combining lignin with other polymers can produce composite materials that have improved resistance to high temperatures. For instance, lignin can enhance its processing capacity, mechanical characteristics, and phosphorescent effectiveness when combined with synthetic polymers or biopolymers. We can customize these composite materials to meet the specific needs of various applications, from bioimaging to environmental sensing.
Nanostructuring and Surface Engineering:
Changing lignin’s structure at the nanoscale level or changing the way its surface behaves might also make it better at giving off red thermally-activated phosphorescence (RTP). Researchers can improve the efficiency of energy absorption and emission, resulting in brighter and more durable phosphorescence, by either adding nanoscale structures or altering the surface chemistry of lignin. Nanostructuring can enhance the stability of lignin RTP materials, rendering them more resilient to environmental influences.
Chemical alterations:
Chemical alterations, such as including functional groups or cross-linking agents, might further augment the thermal resistance qualities of lignin. These alterations have the potential to change the electronic structure of lignin, thereby enabling novel routes for energy absorption and emission. For instance, the addition of electron-withdrawing groups can enhance the energy difference between the singlet and triplet states, resulting in a higher efficiency of phosphorescence.
Possible avenues for advancement in Lignin Modification:
The current study is investigating novel methods to alter lignin to improve its RTP (resistance to thermal degradation) capabilities. These include the creation of hybrid materials that combine lignin with other phosphorescent chemicals, as well as the use of sophisticated computational techniques to develop lignin derivatives with improved properties. The objective is to develop lignin-based RTP materials that possess both sustainability and environmental friendliness while also demonstrating great efficiency and versatility.
An Analysis of Lignin RTP in Comparison to Other Phosphorescent Materials:
When evaluating lignin RTP in comparison to other phosphorescent materials, it is important to take into account many criteria, such as efficiency, sustainability, cost, and environmental impact.
The Real-Time Transport Protocol (RTP) is efficient:
Typically, certain synthetic phosphors specifically designed for high-performance applications outperform lignin in terms of relative thermal performance efficiency (RTP). Nevertheless, chemical alterations and processing methodologies can enhance the effectiveness of lignin RTP. Despite the superior initial performance of synthetic phosphors, the RTP efficiency of lignin can be comparable in situations that prioritize sustainability and biodegradability.
Metrics for measuring sustainability:
Lignin RTP exhibits superior sustainability in comparison to synthetic phosphors. Unlike most manufactured phosphors that derive from finite resources and potentially harm the environment, Lignin is a sustainable and biodegradable substance. Lignin RTP often has a reduced environmental footprint, making it a more sustainable option for applications that prioritize environmental concerns.
Cost-Benefit Analysis:
From a financial standpoint, lignin RTP is more cost-effective than numerous artificial phosphors. Lignin’s affordability, combined with its abundant availability as a byproduct of the paper and pulp industry, makes it a compelling choice for a wide range of uses. Although synthetic phosphors may provide superior performance, their increased expense and negative environmental effects make lignin RTP a more economically viable and environmentally friendly alternative in many situations.
Long-Term Performance:
Considering the durability and longevity required for some applications, the long-term performance of lignin RTP materials is a critical factor to consider. Under specific circumstances, synthetic phosphors may provide superior long-term stability, but chemical changes and protective coatings can enhance the lifetime of lignin RTP materials. To achieve optimal results, it is crucial to strike a harmonious equilibrium between efficiency and eco-friendliness. We must tailor lignin RTP materials to meet individual application requirements while maintaining environmental objectives.
Possibilities for Advancement in Lignin RTP:
The potential for innovation in lignin RTP is immense, with abundant prospects for interdisciplinary research and collaboration.
Current Developments in Phosphorescence Research:
Lignin RTP is leading the way in the study of phosphorescence, with a specific focus on creating sustainable and eco-friendly products. Scientists are investigating innovative methods to improve the thermal resistance qualities of lignin, such as employing advanced nanomaterials, hybrid composites, and unique chemical alterations. These advancements can generate lignin-derived materials that surpass conventional phosphors in terms of both effectiveness and environmental friendliness.
Interdisciplinary Research:
Expertise in various fields, such as material science, chemistry, biology, and environmental science, is necessary for the creation of lignin RTP materials. Interdisciplinary study is crucial for comprehending the fundamental mechanisms of lignin phosphorescence, devising novel processing strategies, and investigating possible applications. Partnerships between academic institutions, businesses, and government organizations can expedite the progress and marketization of lignin RTP materials.
Implementing integration in smart materials and devices:
A diverse array of intelligent materials and systems can incorporate lignin-based materials with thermoplastic properties. For instance, we can employ them in adaptive materials that change their color or emit light in response to environmental stimuli like temperature, pH, or chemical exposure. Various domains, such as environmental monitoring and medical diagnostics, have the potential to use these intelligent materials.
There is potential for collaborative research:
The collaborative research potential in lignin RTP goes beyond the scope of regular academic research. Industry alliances, publicly funded projects, and global collaborations can all contribute to the progress of the area. For instance, collaborations among manufacturers of lignin, scientists specializing in materials, and consumers could facilitate the advancement of lignin RTP materials customized to meet specific market demands. Government support for research and development in sustainable materials could also provide the necessary financing and resources to expedite progress.
Commercialization prospects:
The commercialization of lignin RTP materials holds great potential, especially as the industry is actively searching for sustainable alternatives to synthetic phosphors. To achieve successful commercialization, it is crucial to address the technical obstacles related to lignin RTP. These problems include managing the variability in lignin structure, controlling phosphorescence, and ensuring long-term stability. By tackling these obstacles and showcasing the worth of lignin RTP materials in practical situations, scientists and industry collaborators can facilitate the introduction of lignin RTP into the market and aid in the advancement of a more environmentally friendly future.
Potential Areas for Future Research:
As the area of lignin RTP progresses, several important research directions are expected to influence its future.
Improving Real-Time Transport Protocol (RTP) Efficiency:
One of the main objectives for future studies is to improve the efficiency of RTP (rapid thermal processing) for lignin. This will entail the creation of novel chemical alterations, investigation into the application of cutting-edge nanomaterials, and refinement of processing methods. Enhancing the efficiency of RTP (room temperature phosphorescence) can improve the competitiveness of lignin-based materials compared to synthetic phosphors. This can lead to the exploration of new applications in fields including bioimaging, sensing, and energy harvesting.
Investigating innovative uses:
Future research will also prioritize exploring innovative uses for lignin RTP materials. Potential applications encompass advancements in medicine, such as medication delivery systems or diagnostic instruments, as well as utilization in environmental monitoring, agriculture, and energy storage. Lignin RTP materials have a high degree of adaptability, rendering them appropriate for a diverse array of applications. We anticipate that ongoing research will uncover additional potential applications.
Comprehending the Basics of Chemistry:
Gaining a deeper understanding of the underlying chemistry behind lignin phosphorescence is critical for the advancement of this research. This includes an examination of the electrical composition of lignin, the processes involved in the creation of triplet states, and the variables that affect RTP. Scientists acquiring a deeper understanding of the fundamental chemistry involved, scientists can devise more efficient approaches to improve the thermal stability qualities of lignin.
Increasing Production Capacity:
Another crucial area of emphasis is the expansion of lignin RTP material manufacturing from the laboratory to the industrial scale. This will entail enhancing extraction and processing techniques, devising streamlined manufacturing procedures, and addressing regulatory mandates. Guaranteeing successful production expansion will be critical in making lignin RTP materials available in the market and ensuring their general acceptance.
Ensuring the continued viability and durability over an extended period is crucial:
Future research must prioritize the long-term sustainability of lignin RTP materials. This involves performing thorough life cycle assessments, examining the environmental effects of lignin RTP materials, and investigating strategies to reduce their carbon footprint. By giving priority to sustainability, researchers can create lignin RTP materials that fulfill current requirements without jeopardizing the potential of future generations to fulfill their demands.
In conclusion:
The discovery of photoactivated room-temperature phosphorescence coming from lignin is a big step forward in the study of phosphorescent substances. The distinctive blend of sustainability, cost-efficiency, and eco-friendliness exhibited by lignin renders it a compelling substitute for conventional synthetic phosphors. Despite the challenges of regulating phosphorescence and ensuring long-term stability, current research is actively resolving these issues and preparing lignin RTP materials for market entry.
As research advances, the range of potential uses for lignin RTP materials is growing, encompassing areas such as bioimaging, environmental monitoring, energy harvesting, and smart materials. The prospects for lignin RTP are promising, with abundant possibilities for innovation, interdisciplinary research, and economic exploitation. Scientists and industry collaborators may develop eco-friendly materials that possess exceptional performance characteristics, making a significant contribution towards a more sustainable future by harnessing the inherent abundance and adaptability of lignin.
Frequently Asked Questions:
1). What distinguishes lignin as a phosphorescent material?
Due to its natural abundance, sustainability, and complicated chemical structure, lignin is highly suitable for phosphorescent applications. The capacity to demonstrate photoactivated room temperature phosphorescence (RTP) provides a sustainable substitute for synthetic phosphors.
2). What is the photoactivation mechanism in RTP?
In RTP, photoactivation involves subjecting a substance to light, which stimulates its electrons to ascend to higher energy states. During the lignin RTP, the electrons become trapped in a metastable triplet state, gradually transitioning back to the ground state and producing light over time.
3). What are the primary obstacles in the development of lignin RTP?
The primary obstacles include regulating phosphorescence, managing heterogeneity in lignin structure, ensuring stability at ambient temperature, and expanding production for commercial applications.
4). Is it possible to utilize lignin RTP in common consumer goods?
Lignin RTP exhibits considerable promise for utilization in various common products, such as bioimaging probes, environmental sensors, and smart materials. The sustainability and biodegradability of this material make it a desirable choice for consumer applications.
5). What are the likely environmental consequences of widespread lignin RTP usage?
The environmental impact of lignin RTP is predominantly beneficial, given that lignin is a renewable and biodegradable substance. Nevertheless, it is crucial to analyze the decomposition byproducts of lignin RTP materials to do not pose any negative impacts on the environment or human health.
For more chemistry blogs, visit chemistry Master