Table of Contents
Overview of Photoredox Catalysis:
Carbon-carbon (C-C) cross-coupling reactions are fundamental in contemporary organic synthesis, facilitating the creation of intricate molecules from less complex starting materials. These reactions are essential in the pharmaceutical industry, agrochemicals, and materials research. Conventional approaches frequently depend on palladium (Pd) catalysts, which, although efficient, have drawbacks including exorbitant expense, toxicity, and susceptibility to reaction circumstances. Photoredox catalysis is a very effective alternative that harnesses light to facilitate chemical reactions, providing gentler reaction conditions and improved efficiency. Recently, in this field, Au(I) complexes with two-coordinated structures have emerged as catalysts that show great promise. This article examines the function of these complexes in photoredox catalysis for C-C cross-coupling processes, emphasizing their methods, benefits, applications, and prospects. Photoredox catalytic C(Ar)–C(Ar) cross-coupling reactions.
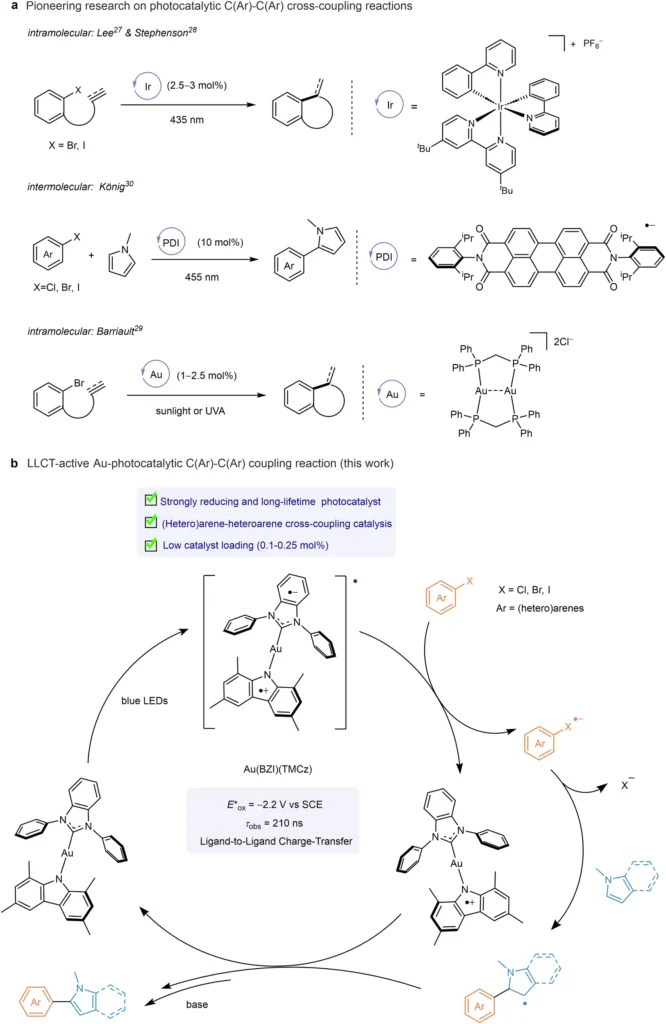
Comprehending the Mechanisms of Photoredox Catalysis:
Definition and fundamental principles:
Photoredox catalysis is a mechanism that uses light to activate a photocatalyst, allowing electrons to flow between substrates and initiate chemical processes. Light energy stimulates the photocatalyst, generating an excited state that can engage in redox processes. This technique uses light energy, typically from visible or ultraviolet (UV) sources, to facilitate reactions that may be difficult to achieve with heat alone.
Historical Context:
Since the early investigations into photochemistry, the use of light to initiate chemical reactions has been documented. Photoredox catalysis emerged as a formalized discipline in the early 21st century. Significant achievements include the advancement of ruthenium (Ru) and iridium (Ir) complexes as highly effective photocatalysts. These complexes have facilitated a range of organic transformations, such as C-C cross-coupling reactions, by providing excellent efficiency and selectivity at low temperatures.
Primary Benefits Compared to Conventional Approaches:
Photoredox catalysis provides numerous benefits compared to conventional thermal or metal-catalyzed reactions:
Gentler Conditions: Reactions can occur at room temperature and pressure, thereby reducing the need for severe conditions.
Light enables high selectivity by providing precise regulation of reaction pathways, hence limiting the formation of by-products.
Energy Efficiency: Utilizing light, especially visible light, can enhance energy efficiency by reducing energy usage in chemical processes.
Environmental Advantages: Decreased dependence on toxic and costly metals such as Pd and the utilization of less dangerous substances conform to the principles of green chemistry. Catalysis cycle.
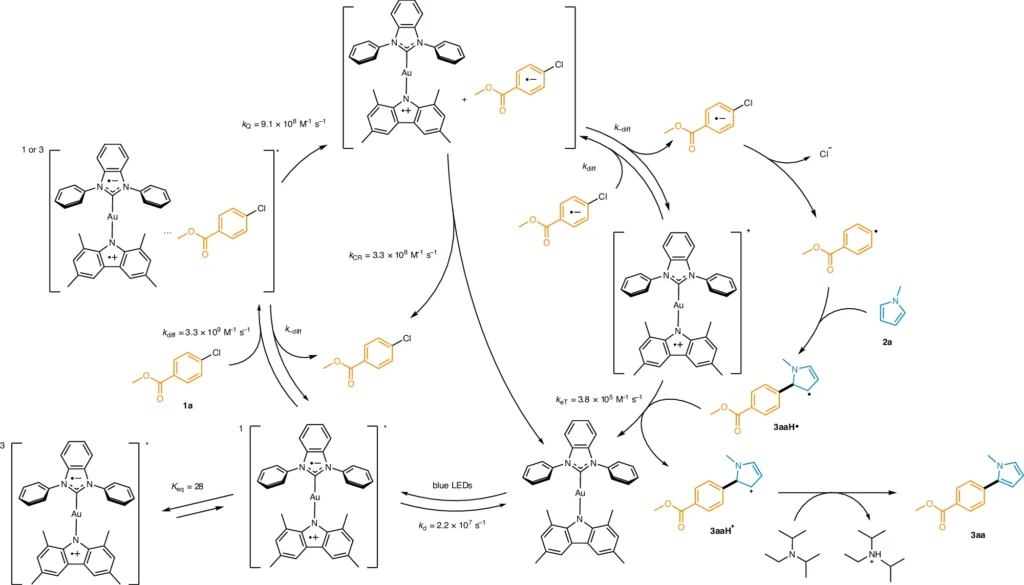
C-C cross-coupling reactions:
Organic Synthesis: Definition and Importance
C-C cross-coupling reactions entail the creation of a chemical bond between two organic molecules, namely carbon-carbon bonds. These reactions are essential for the formation of intricate organic structures and play a critical role in the production of medicines, agrochemicals, natural goods, and sophisticated materials. By creating C-C bonds, chemists can construct complex molecular structures that are essential for biological function and material characteristics.
Conventional catalysts and their constraints:
Historically, people have widely regarded Pd-catalyzed cross-coupling reactions, such as the Suzuki-Miyaura, Heck, and Negishi couplings, as the most reliable and effective methods. Although these strategies are highly effective, they encounter certain challenges:
Cost and Availability: Due to its high cost and limited geographic availability, palladium is a costly metal for large-scale applications.
Toxicity: Palladium (Pd) and its compounds have the potential to be toxic, presenting both environmental and health hazards.
Reaction Conditions: Palladium-catalyzed reactions typically necessitate elevated temperatures and pressures, as well as delicate ligands and reagents.
Compatibility Limitations: The incompatibility of Pd catalysis with specific functional groups and substrates restricts its use.
The role of photoredox catalysis in enhancing these reactions:
Photoredox catalysis overcomes numerous constraints associated with conventional approaches. Photoredox Catalysis uses light to activate catalysts, allowing them to function at lower temperatures and pressures, thereby decreasing the need for significant thermal energy and force. This not only enhances the safety and mitigates the environmental impact of the reactions, but also expands the spectrum of substances suitable for use, including those with delicate functional groups. Moreover, employing non-toxic, readily available metals as photocatalysts presents a more environmentally friendly substitute for Pd.
Catalysis Utilizing Au(I) Complexes:
An Overview of Gold Catalysis:
In recent years, there has been a renewed interest in the use of gold as a catalyst, especially with the utilization of Au (I) and Au (III) complexes. Gold, formerly believed to be chemically unreactive, has exhibited exceptional catalytic characteristics, particularly in the field of organic transformations. Au(I) complexes have demonstrated effectiveness in promoting various processes, such as oxidations, reductions, and C-C couplings.
Distinctive Characteristics of Au(I) Complexes:
Au(I) complexes possess several features that make them highly desirable for catalytic purposes:
Stability: Au(I) complexes possess exceptional stability when subjected to different reaction conditions, hence impeding breakdown and guaranteeing constant performance.
Electronic Properties: Gold’s distinct electronic arrangement enables it to stabilize reactive intermediates, allowing for intricate reaction paths.
Catalytic Efficiency: Au-I complexes have remarkable catalytic efficiency, as evidenced by their ability to achieve high turnover numbers (TONs) and turnover frequencies (TOFs).
Mild Reaction Conditions: Gold catalysts often function under less severe reaction conditions in comparison to other metals, resulting in lower energy requirements and enhanced safety.
Comparative analysis of metal catalysts:
Although Pd is often considered the standard for many catalytic processes, Au(I) presents numerous distinct advantages:
Cost and Availability: Despite its preciousness, catalysis frequently uses gold at lower loadings, making it more economically viable in some applications.
Toxicity: Gold exhibits lower toxicity compared to palladium, resulting in reduced environmental and health hazards.
Reactivity: Au(I) complexes have distinctive reactivity, enabling the initiation of reaction pathways that are not attainable with Pd or other metals. This feature opens up novel synthetic opportunities. Photoreducing ability.
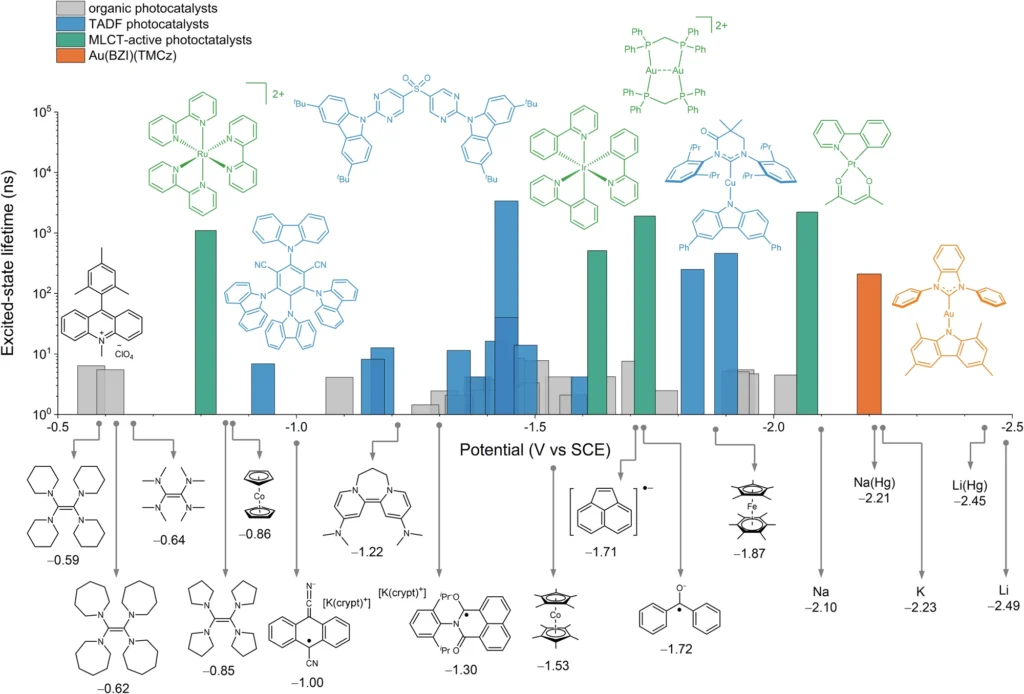
The coordination number of Au(I) is two:
The structure’s characteristics:
The uncomplicated coordination environment of Au(I) complexes with a coordination number of two, typically linking a gold atom to two ligands, distinguishes them. The ligands can exist in a neutral state, such as phosphines, or in an anionic state, such as halides or carbenes. This coordination environment’s straightforwardness enhances the complexes’ strong reactivity and stability.
Methods of synthesis:
The formation of two-coordinated Au(I) complexes is typically uncomplicated, requiring the reaction of gold precursors (such as AuCl) with suitable ligands. Phosphines, carbenes, and alkyl or aryl groups are often encountered as ligands. The selection of ligands can greatly impact the characteristics and reactivity of the resultant complex, enabling customization according to the desired catalytic use.
Stability and reactivity:
Au(I) complexes with two coordination sites demonstrate exceptional stability when subjected to different reaction circumstances, such as exposure to air and moisture. The electronic and steric characteristics of the ligands determine their reactivity, with ligands that donate electrons increasing catalytic activity. The combination of stability and reactivity in these complexes makes them very suitable for photoredox catalysis, where maintaining constant performance and achieving high efficiency are of utmost importance. Oxidative quenching.
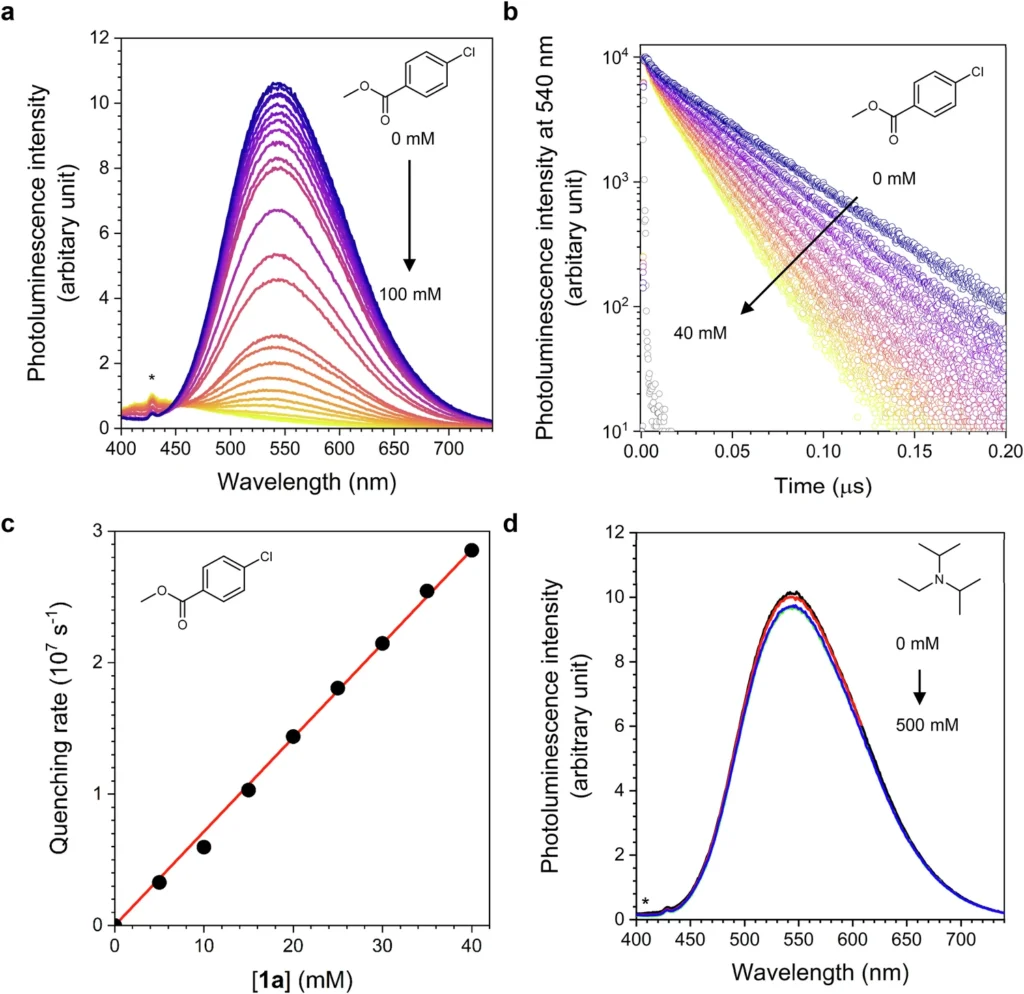
Photoredox catalysis operates through a specific mechanism of action:
Photon Absorption and Stimulated Emission:
Photoredox catalysis involves the absorption of light by a two-coordinated Au (I) complex, usually in the visible or UV range. The absorption of light causes the complex to enter an excited state, enabling it to engage in redox processes. The efficiency of these catalysts is heavily dependent on their ability to effectively absorb light, as this factor governs the start and development of the catalytic cycle.
Electron transfer processes:
When the Au(I) complex is excited, it can help transport electrons between substrates. This process entails the movement of an electron from a molecule that donates it to a molecule that accepts it, facilitated by the Au(I) complex. Electron transfer can trigger the creation of highly reactive intermediates, such as radicals or ion pairs, which subsequently undergo further reactions to produce the intended products.
The function of the Au(I) complex in the catalytic cycle:
The Au(I) complex has a crucial function in the catalytic cycle, serving as both an electron mediator and a stabilizing agent for reactive intermediates. The process often entails:
Excitation occurs when the Au(I) complex absorbs light and transitions to an excited state.
Electron Transfer: Enhancing the movement of electrons between substances leads to the creation of highly reactive intermediate compounds.
Bond formation occurs when the intermediates react to create a C-C bond, causing the Au(I) complex to return to its original state and become available for another cycle.
This process ensures a high level of catalytic efficiency by constantly renewing and involving the Au(I) complex in several reaction cycles.
Benefits of Utilizing Two-Coordinated Au(I) Complexes:
Improved Catalytic Efficiency:
Au(I) complexes with two coordination sites demonstrate exceptional catalytic efficacy, resulting in accelerated reaction kinetics and increased product yields. Due to their capacity to efficiently absorb light and facilitate electron transfer processes under moderate circumstances, they surpass numerous conventional catalysts.
Enhancements in Selectivity and Yield:
These complexes exhibit exceptional selectivity, minimizing the generation of by-products and enhancing the intended product’s production yield. The ability to precisely manipulate the progression of chemical reactions through photoredox catalysis, in conjunction with the distinct characteristics of Au(I) complexes, guarantees a high degree of selectivity.
Advantages in terms of the environment and economy:
Au(I) complexes have lower toxicity compared to numerous other metal catalysts, such as Pd, when considering their environmental impact. Their use adheres to green chemistry principles, reducing dependence on perilous reagents and severe conditions. From an economic standpoint, although gold is a valuable metal, the exceptional efficiency and minimal loading needs of Au(I) complexes can compensate for the initial expense, rendering them a practical choice for large-scale applications. Electron transfer.
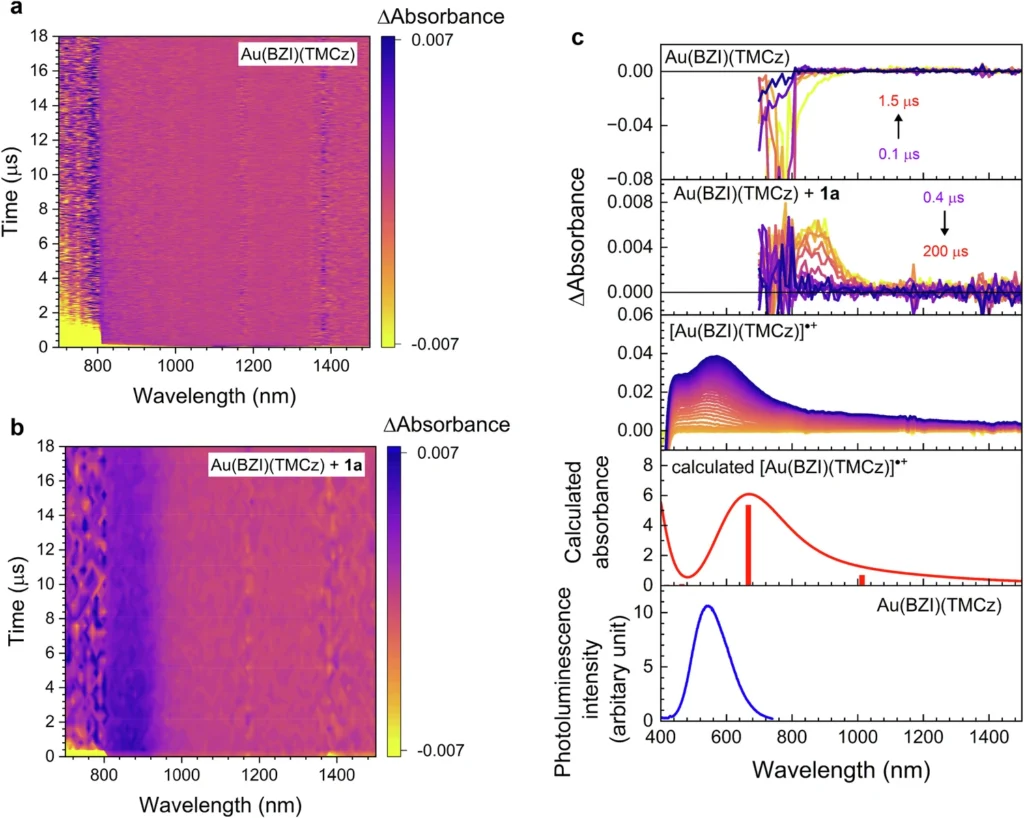
Analysis of specific situations and practical implementations:
Illustrations of effective C-C cross-coupling reactions:
Multiple case studies demonstrate the efficacy of two-coordinated Au(I) complexes in C-C cross-coupling processes. An example of this is the joining of aryl halides with organoboron compounds, which demonstrated exceptional effectiveness and specificity when facilitated by Au(I) complexes. These reactions often occur at low temperatures and produce a large amount of desired products while generating only a small amount of unwanted by-products.
Another example involves the successful use of Au(I)-catalyzed cross-coupling of alkynes and alkenes to synthesize intricate natural compounds. Precise control over the reaction environment, facilitated by the use of light as a driving force, has allowed for the creation of molecules with complex structural characteristics.
Comparative analysis of alternative catalytic systems:
Au(I) complexes have various advantages in comparison to standard Pd-catalyzed systems:
Reduced reaction temperatures: Numerous processes catalyzed by Au(I) occur at room temperature, resulting in decreased energy requirements.
Enhanced Substrate Scope: Au(I) complexes have an enhanced substrate scope, as they are capable of accommodating a wider variety of functional groups and substrates. This expands the spectrum of reactions that can be performed.
Reduced Toxicity: Gold (I) catalysis is more environmentally friendly and safer for industrial uses due to its decreased toxicity compared to palladium (Pd).
Industrial Applications and Scalability:
Another notable benefit of Au(I) catalysis is its scalability. Au(I) complexes have been effectively incorporated into several industrial processes, showcasing their viability for large-scale production. For example, the pharmaceutical industry has included Au(I)-catalyzed cross-coupling processes in the production of active pharmaceutical ingredients (APIs), taking advantage of the catalysts’ exceptional efficiency and selectivity.
Obstacles and Constraints:
Limitations of Au(I) Catalysis:
Although Au(I) catalysis offers numerous benefits, it also encounters certain obstacles:
High Cost of Gold: The high cost of gold is a significant factor to consider, especially for large-scale applications, notwithstanding the potential for using Au(I) complexes at modest loadings.
Ligand Design: The effectiveness of Au(I) complexes relies heavily on the selection of ligands. Developing ligands that achieve an optimal equilibrium between stability and reactivity is a challenging task that necessitates thorough investigation.
Stability Under Various Conditions: Au(I) complexes exhibit stability, although their performance may vary depending on the specific reaction conditions. Ensuring consistent performance in many settings is essential for achieving universal acceptance.
Technical and economic obstacles:
To tackle the technical and economic difficulties related to Au(I) catalysis, various approaches might be employed:
Cost Reduction: The development of techniques to recycle and reuse Au(I) complexes can effectively alleviate the exorbitant expenses associated with gold. Furthermore, improvements in ligand design have the potential to optimize the effectiveness of Au(I) catalysts, resulting in a decrease in the quantity needed for reactions.
Improved Stability: Investigating novel ligands and complex architectures can enhance the stability of Au(I) complexes, guaranteeing consistent performance across diverse environments.
Scalability: Ongoing endeavors to enhance reaction conditions and expand procedures will be essential for the practical implementation of Au(I) catalysis. Conducting pilot studies and establishing industrial alliances can facilitate the transition from laboratory research to commercial applications. Electron transfer kinetics.
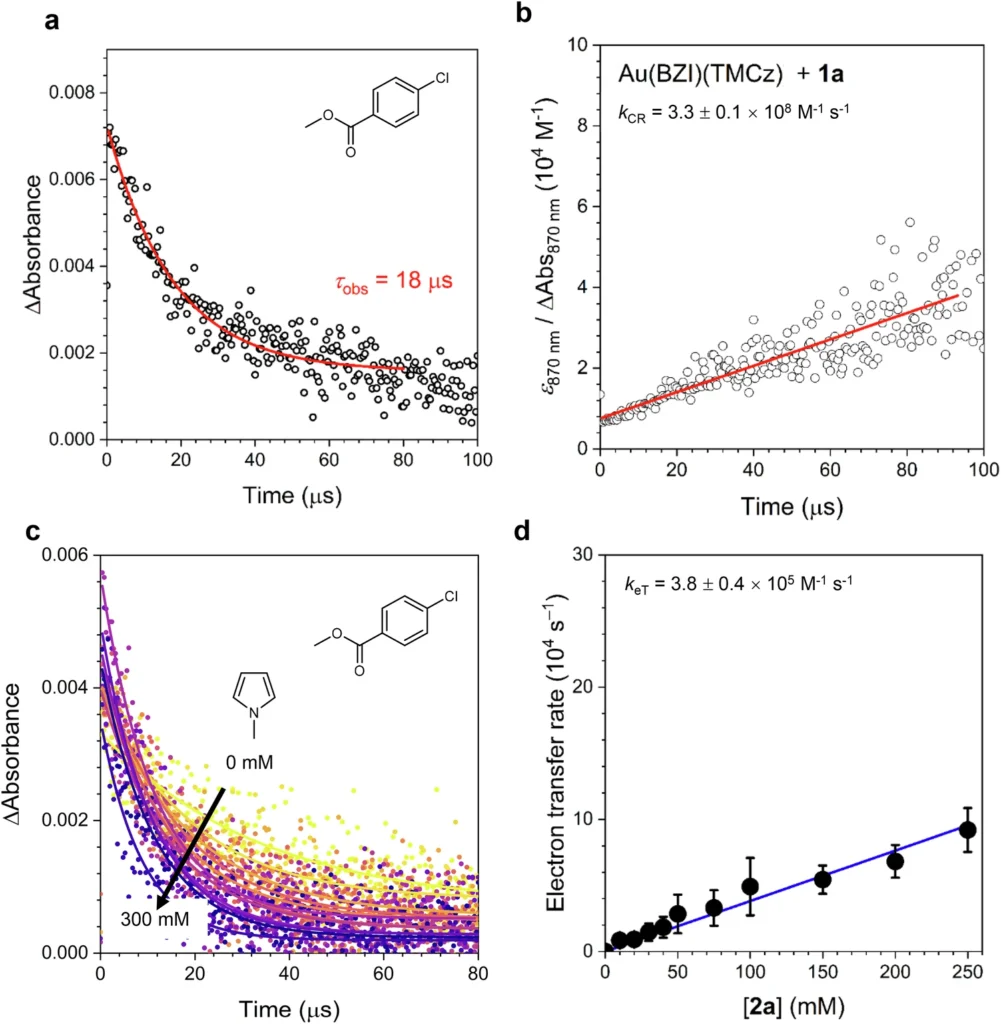
Prospects for Advancements in Photoredox Catalysis:
Current developments and advancements in technology:
The prospects for the application of two-coordinated Au(I) complexes in photoredox catalysis are promising, with the emergence of various trends and technologies.
New Ligand Designs: Researchers are investigating innovative ligand designs to augment the characteristics of Au(I) complexes, hence enhancing their efficiency, selectivity, and stability.
Hybrid Catalytic Systems: The integration of photoredox catalysis with other catalytic processes, such as enzymatic or electrochemical catalysis, might result in the formation of hybrid systems that possess improved capabilities.
Advanced light sources, such as highly efficient LEDs and laser systems, have the potential to enhance the energy efficiency and precision of photoredox reactions.
Possibilities for Novel Catalyst Designs
Au(I) complexes have the potential to improve performance through innovative catalyst designs:
Bimetallic Systems: Bimetallic complexes can be formed by combining Au(I) with other metals, resulting in synergistic features that provide novel reactivity and selectivity profiles.
Designing nanostructured Au(I) catalysts can enhance their surface area and reactivity, hence improving catalytic efficiency.
Photoresponsive Ligands: By including ligands that are responsive to light and undergo changes in characteristics upon exposure, it is possible to achieve dynamic control over catalytic activity. This allows for the ability to modify reactivity as needed.
Integration with Other Catalytic Processes:
The combination of photoredox catalysis with other catalytic processes has intriguing prospects.
Electrophotocatalysis combines photoredox catalysis and electrochemical methods to make electrophotocatalytic devices that work very well. This synergistic approach enables the exploration of novel reaction pathways and significantly improves overall efficiency.
Bioorthogonal catalysis combines photoredox catalysis with biological systems. This makes it possible for bioorthogonal reactions that increase the number of chemical changes that can happen in biological settings.
Flow chemistry approaches can enhance the scalability and reaction control of photoredox processes, making them more appropriate for industrial applications.
In conclusion:
Photoredox Catalysis, Au(I) complexes with two-coordinated structures have had a considerable influence on photoredox catalysis, especially in C-C cross-coupling processes. Their distinct characteristics, combined with the advantages of photoredox techniques, provide a potent instrument for organic synthesis. These compounds offer improved catalytic efficiency, selectivity, and yield, as well as environmental and economic advantages.
Although there are obstacles to overcome, such as the expensive nature of gold and the requirement for additional investigation into ligand design and stability, the outlook for Au(I) catalysis is optimistic. As catalyst design, light source technology, and the combination of photoredox catalysis with other catalytic processes continue to improve, it will be easier to make catalytic systems that work better, are more specific, and are better for the environment.
Frequently Asked Questions:
1). C-C cross-coupling reactions refer to chemical reactions where two carbon atoms are connected to form a new carbon-carbon bond.
C-C cross-coupling reactions are a crucial process in which carbon-carbon bonds are formed between two organic compounds. This process plays a vital role in constructing intricate molecular structures for medicines, agrochemicals, and materials science.
2). What is the significance of photoredox catalysis?
Light is used to activate catalysts in photoredox catalysis, which offers safer reaction conditions, better selectivity, and higher yields than traditional methods. It adheres to the principles of green chemistry by decreasing the dependence on harsh chemicals and conditions.
3). What are the factors that contribute to the effectiveness of Au(I) complexes in catalysis?
Au(I) complexes are very useful because they have unique electrical properties, are strong, and can allow electron transport mechanisms to work. Because these complexes can stabilize highly reactive intermediates and take part in redox processes, they make the reaction more efficient and selective as a whole.
4). What advantages are associated with using two-coordinated Au(I) complexes?
Au(I) complexes with two coordination sites exhibit improved catalytic efficiency, selectivity, and yield. They function under less severe conditions, minimize the production of secondary substances, and are advantageous in terms of both environmental and economic factors because of their stability and utilization of light energy.
5). What are the problems associated with Au(I) catalysis?
Challenges include the exorbitant cost of gold, the need for meticulous ligand design, and the assurance of stability for Au(I) complexes across a variety of reaction circumstances. To tackle these problems, it is necessary to devise economical recycling techniques, improve the durability of catalysts, and optimize the reaction parameters for widespread use.
For more chemistry blogs, visit chemistry Master