Table of Contents
Polymerization, the act of connecting monomers to create polymers, is a fundamental aspect of contemporary chemistry and materials research. Superbase-mediated selective polymerization is a highly effective technique for producing polymers with precise structures and characteristics, making it a valuable method in polymer synthesis. Researchers have achieved notable progress in this sector in recent years, specifically through the creation of proton-triggered topological transformations. This improvement allows for the production of cyclic polymers with extremely high molar masses.
An overview of proton-triggered topological transformation:
a). Definition and significance: Polymerization is a widespread phenomenon in nature, manifesting in various processes such as the assembly of DNA molecules and the production of plastics and rubbers. A significant obstacle in polymerization is the regulation of the structure and characteristics of the resultant polymer is a significant obstacle to polymerization. Conventional polymerization techniques frequently produce polymers that are either linear or branched, and these polymers may not exhibit the desired characteristics for specific applications.
b). A brief overview of selective polymerization:
The utilisation of proton-triggered topological transformation presents a new and innovative method for polymer synthesis. This technique enables the precise creation of cyclic polymers with extremely high molar weights. This technique entails the deliberate reorganisation of polymer chains by the impact of protons, leading to the creation of closed-loop structures. Researchers can manipulate the structure of polymers by utilising the responsiveness of superbases and the catalytic strength of protons. This allows for exact control over the arrangement of the polymers, resulting in materials that possess distinct features and functionalities.
c). Importance of ultrahigh-molar-mass cyclic polymers:
The significance of proton-triggered topological transformation lies in its ability to reach a category of polymers that were previously difficult to produce. Ultrahigh-molar-mass cyclic polymers offer remarkable mechanical strength, thermal stability, and resistance to degradation, making them ideal for a wide range of applications such as advanced materials, drug delivery systems, and biomaterials.
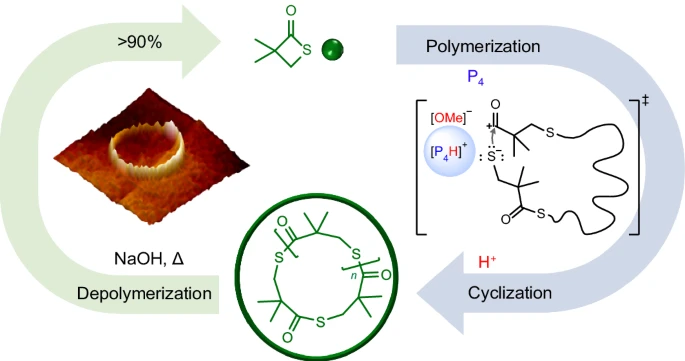
An Exploration of Suprabases in Polymerization:
a). Explanation of superbases: Superbases are a category of extremely alkaline chemicals that have a vital function in promoting polymerization processes. These chemicals exhibit the capacity to remove a hydrogen ion from a diverse array of substances, rendering them efficient catalysts for polymerization reactions. Superbases can start and spread polymerization reactions by removing protons from individual units, resulting in the creation of polymer chains.
b). Mechanism of superbase-mediated polymerization:
The process of superbase-mediated polymerization comprises multiple stages, which include initiation, propagation, and termination. During the initiation process, the highly basic catalyst removes a proton from the individual feedstock molecule, creating reactive species that are capable of undergoing polymerization. A sequence of propagation phases continues the polymerization reaction, incorporating monomeric units into the developing polymer chain. Ultimately, the reaction concludes either through the utilisation of individual units or through the inactivation of the catalyst.
c). Types of superbases and their applications:
Researchers have examined the effectiveness of several categories of highly basic compounds in polymerization reactions, including alkali metal amides, phosphazenes, and organolithium compounds. These compounds have clear benefits in terms of their ability to react, their ability to selectively react with specific substrates, and their ability to work well with various monomeric substrates. Due to their wide substrate scope, alkali metal amides exhibit significant reactivity and can effectively participate in a diverse array of polymerization reactions.
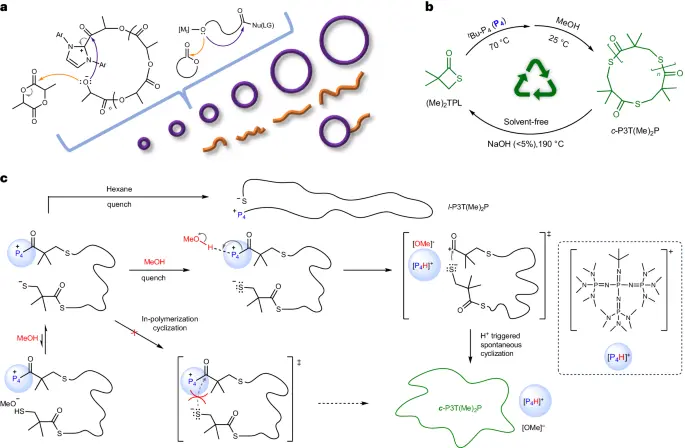
Proton-induced Topological Transformation Process:
a). Detailed steps of the transformation process: The proton-triggered topological transformation process consists of multiple critical phases, each of which is necessary for the successful production of ultrahigh-molar-mass cyclic polymers. The method begins by introducing a highly basic catalyst into the monomeric feedstock to initiate polymerization. The superbase catalyst removes the hydrogen atoms from the individual units, creating reactive species that are capable of undergoing polymerization.
b). Factors influencing the transformation:
The process of topological transformation initiated by protons is characterised by a high level of selectivity, enabling precise control over the topology of polymer chains and their molecular weight. Researchers can customise the characteristics of the resultant polymers to satisfy specific needs by precisely adjusting reaction variables such as temperature, pressure, and catalyst concentration. Using advanced analytical techniques like mass spectrometry and nuclear magnetic resonance spectroscopy also lets us look closely at the synthesised polymers, giving us useful details about their structure and properties.
c). Examples of proton-triggered topological transformation reactions:
Cyclic polyethene glycol (PEG) polymers and cyclic polystyrene (PS) polymers undergo proton-triggered topological transformation processes during their synthesis. During these reactions, the superbase catalyst takes away a proton from the hydroxyl or aromatic groups of the individual units. This makes reactive intermediates that can go through intramolecular cyclization. The resulting cyclic polymers have remarkable characteristics, such as elevated molecular weights, precise molecular weight distributions, and regulated topologies.
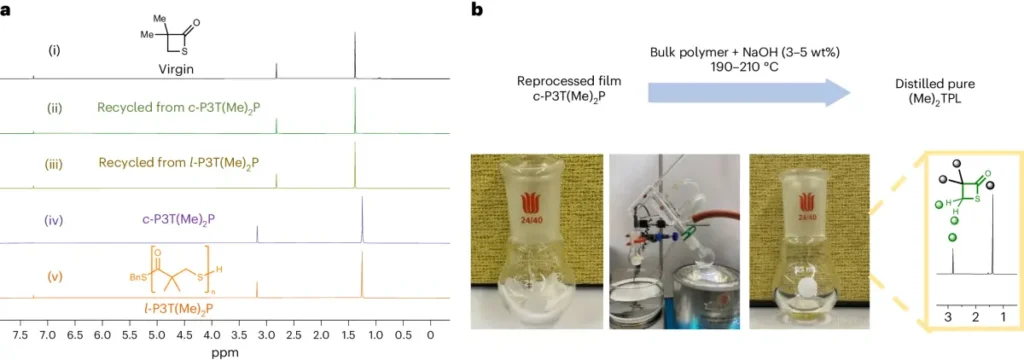
Benefits of proton-triggered transformation:
a). Properties and characteristics of ultrahigh-molar-mass cyclic polymers: One of the best things about proton-triggered topological transformation is that it can make cyclic polymers with very high molecular masses that have specific properties and functions. These polymers display distinctive physical and chemical characteristics, such as remarkable mechanical robustness, thermal endurance, and resistance to deterioration. Ultrahigh-molar-mass cyclic polymers find utility in diverse sectors such as materials science, biology, and nanotechnology, where their exceptional characteristics can be utilised to create cutting-edge materials and products.
b). Applications in various industries:
The proton-triggered topological transformation has several clear advantages when compared to standard polymerization methods. Firstly, the approach enables meticulous regulation of polymer chain topology and molecular weight, leading to polymers with consistent structures and properties. Furthermore, the utilisation of superbase catalysts allows for the production of polymers using gentle reaction conditions, thereby minimising the requirement for aggressive reagents and energy-demanding procedures. Proton-triggered transformation can be readily customised to suit various monomeric substrates and reaction circumstances, rendering it a versatile instrument for polymer synthesis.
c). Comparison with conventional polymerization methods:
Ultrahigh-molar-mass cyclic polymers have a broad range of applications across various sectors and technologies. Within the realm of materials research, these polymers are employed to create top-tier materials such as fibres, films, and coatings that possess extraordinary mechanical properties. Cyclic polymers are used in biotechnology for drug delivery systems due to their desirable characteristics, such as controlled release qualities and biocompatibility, making them well-suited for therapeutic purposes. Cyclic polymers are used in nanotechnology to construct nanoscale devices and structures. Their distinctive characteristics allow for accurate regulation of both structure and function.
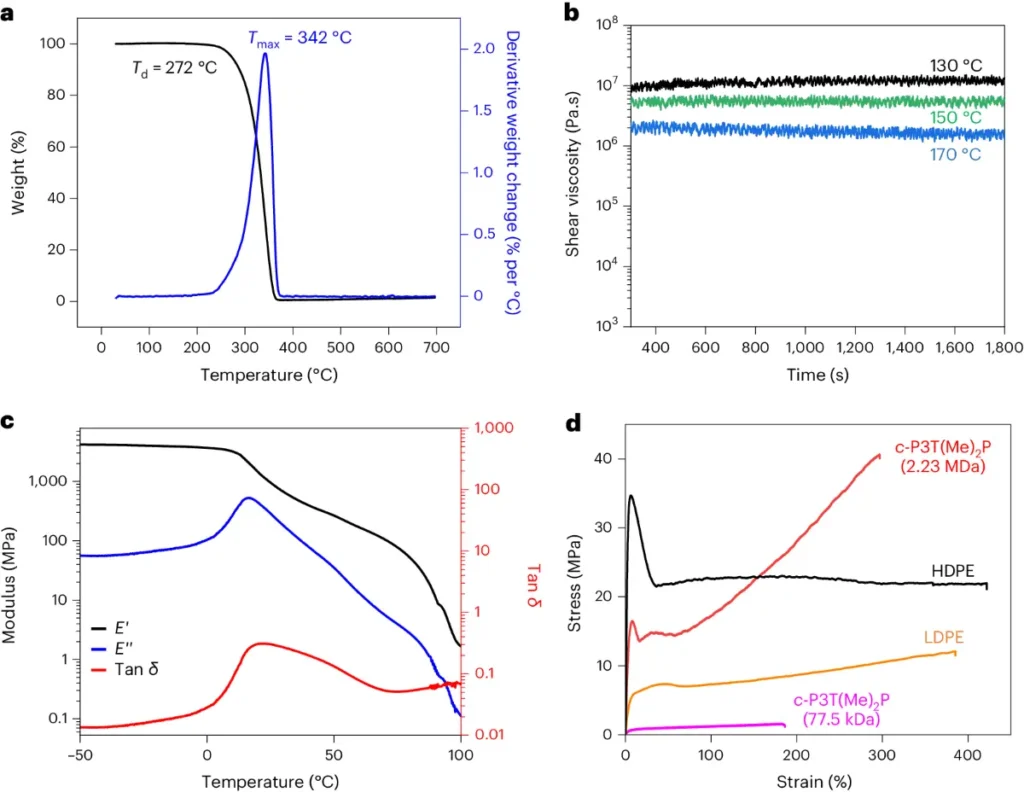
Obstacles and Constraints:
a). Technical challenges in scaling up production: Despite its numerous benefits, the proton-triggered topological transformation process faces various obstacles and restrictions that require resolution to fully utilize its capabilities. An important obstacle is the ability to scale up the process, particularly when it comes to producing ultrahigh-molar-mass cyclic polymers on an industrial scale. Presently, synthetic methods frequently depend on batch reactions carried out in the laboratory, which may not be appropriate for large-scale manufacturing. It is crucial to develop scalable synthetic methods and optimise reaction conditions for industrial applications to successfully commercialise proton-triggered transformation technology.
b). Issues related to catalyst efficiency and selectivity:
Another obstacle is the transformation process’s effectiveness and specificity. Although proton-triggered topological transformation allows for precise manipulation of polymer structure and characteristics, achieving high yields and selectivities can be difficult. Catalyst deactivation, side reactions, and contaminants in the reaction mixture can influence the efficiency of the transformation process. It is crucial to develop strong catalytic systems and reaction procedures that minimise unwanted reactions and contaminants to enhance the efficiency and dependability of proton-triggered transformation processes.
c). Environmental considerations:
When assessing the sustainability of a proton-triggered topological transition, it is crucial to take into account environmental factors. A significant number of catalysts and reagents utilised in the production of ultrahigh-molar-mass cyclic polymers are sourced from fossil fuels and could potentially cause harmful effects on the environment. To reduce the environmental impact of proton-triggered transformation technology, it is critical to focus on developing more environmentally friendly methods of synthesis and using renewable raw materials.
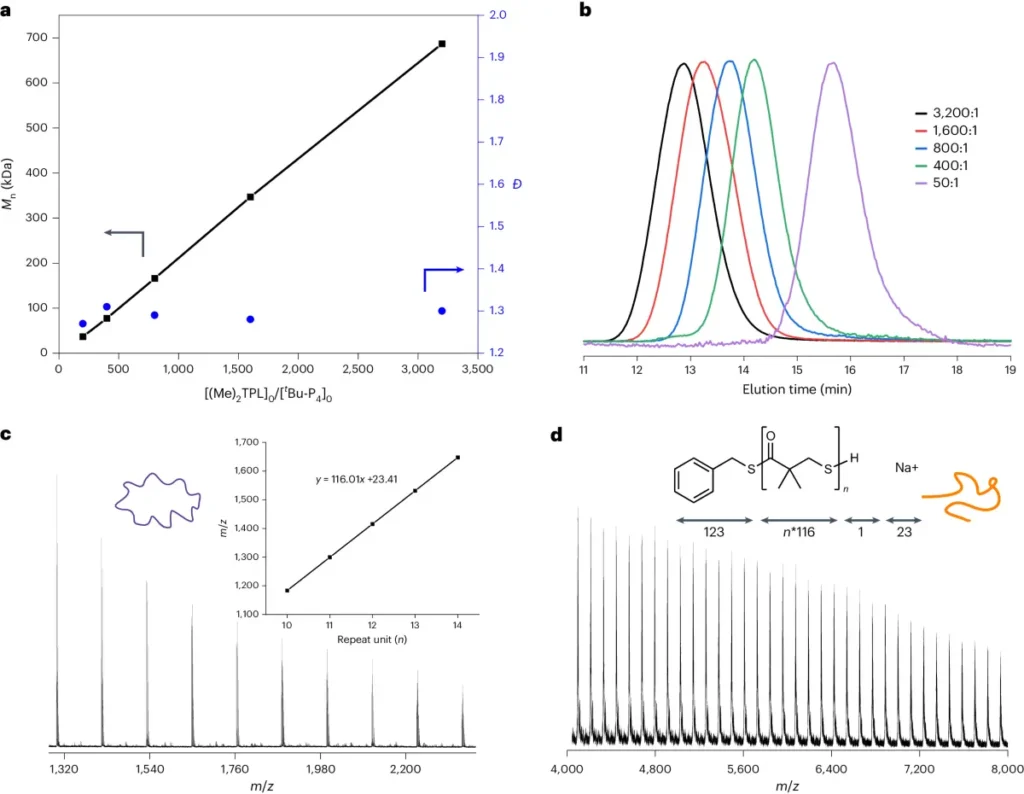
Future Opportunities and Research Directions:
a). Potential advancements in proton-triggered transformation technology: The field of proton-triggered topological transformation has great potential for creating new materials with exceptional characteristics and functionalities. Subsequent research endeavours will likely concentrate on tackling the aforementioned difficulties and advancing the current level of proton-triggered transformation technology.
A promising area of research is the development of novel catalysts and reaction procedures to improve the efficiency and selectivity of proton-triggered transformation processes. Researchers can address the technical problems of synthesising ultrahigh-molar-mass cyclic polymers by creating catalysts that have improved activity and stability.
b). Emerging trends and research areas:
Another avenue of research is investigating interdisciplinary collaborations among chemists, materials scientists, and engineers. By leveraging experience from several areas, researchers can find creative answers to complex challenges in proton-triggered topological change. Collaborative endeavours may encompass the development of novel materials, the enhancement of reaction parameters, and the expansion of production methodologies to cater to commercial applications.
c). Collaborative efforts in academia and industry:
Future research endeavours may also prioritise the examination of the wider consequences of proton-triggered topological transitions for society and the environment, in addition to technological progress. Gaining a comprehensive understanding of the economic, social, and environmental consequences of this technology will be crucial in directing its responsible advancement and implementation.
In conclusion:
Overall, the utilisation of protons to initiate topological transformation offers a revolutionary method for polymer synthesis. This technique allows for the precise creation of cyclic polymers with extremely high molecular weights while also customising their characteristics and functionalities. Researchers have discovered that by utilising the reactivity of superbases and the catalytic capability of protons, they can now explore new possibilities for material innovation in various industries and technologies. Despite certain remaining problems, the future of proton-triggered transformation technology appears to be promising, offering chances for further research, collaboration, and innovation.
Distinct Frequently Asked Questions:
1). What sets proton-triggered topological transformation apart from standard polymerization methods?
Conventional polymerization methods cannot easily create cyclic polymers with extremely large molar masses, but the proton-triggered transformation process offers a distinct route.
2). What are the possible uses of ultrahigh-molar-mass cyclic polymers?
These polymers are used in different sectors, such as materials science, biology, and nanotechnology. Their outstanding qualities are used to create sophisticated materials and gadgets.
3). What obstacles must be overcome to achieve a broad implementation of proton-triggered transformation?
The challenges encompass improving the reaction conditions to make them suitable for large-scale production, increasing the effectiveness of the catalyst, and expanding the range of substrates that can undergo the transformation process.
4). What methods may researchers employ to enhance the specificity of proton-induced topological changes?
Researchers can enhance the selectivity of the transformation process by adjusting reaction parameters, investigating new catalysts, and developing a more comprehensive understanding of the underlying mechanisms.
5). What are the possible environmental consequences of proton-induced topological transformation?
Although proton-triggered transformation presents promising prospects for material innovation, researchers must also take into account the ecological consequences of the process and strive to establish sustainable techniques.
For more chemistry blogs, visit chemistry Master